Maternal effects modulate offspring development and population dynamics in the wild
21 - 01 - 2013
Abstract
Maternal effects (MEs) are defined as the causal influence of the mother genotype or phenotype on her offspring phenotype. As they impact on the individual phenotype, MEs have been increasingly studied in recent years in evolutionary ecology. However, no recent compilation has been made in order to understand MEs under natural conditions. Here, I review the MEs in animal populations from recent works in the field. MEs are of very importance before parturition or hatching and, for altricial species, after the birth. MEs are also capital at a population scale for population dynamic and evolution. Focus will be made on whose fitness MEs mainly increase or lower and, when possible, on the physiological mechanisms underlying such modulators of phenotypic variation.
Table of Contents
Introduction
Evolution is partly driven by natural selection which acts on the fitness of organisms, i.e their longevity and reproductive success. Both fitness components are life history traits that rely on the phenotype of an individual which depends on its genes and its environment. Because in most species mothers provide the developmental environment, evolutionary students have to take into account maternal effects (MEs), which are the causal influence of maternal genotype or phenotype on the offspring phenotype (OP) – for the importance of the causality link, see box 1.
MEs especially operate during early life but can have long-term impact on OP. Some of them tend to increase the offspring fitness whereas other seem to reduce it (see box 2), but the overall outcome fluctuates with the life context [1]••. Altogether, all MEs generally rely on the same mechanisms: prenatal influence on OP is tied with molecular pathways (hormones, mRNA or proteins deposited into the zygotes) whereas postnatal MEs mainly result from maternal care [2]••. Consequently, because MEs are dependent on the link between mothers and offspring, they are of greatest importance in altricial species (where young animals depend on their parents) such as mammals or birds but also in anurans or insects.
Recent development of genetic tools (molecular markers such as microsatellites) has allowed pedigree inference, and new statistical methods now permit quantitative distinction of additive genetic variance and phenotypic variance [3]•. For instance, the animal mixed-effects model, which requires long-time series of the relatedness of individuals in a population, partitions the variance into different genetic and environmental sources to estimate key parameters such as the heritability of a trait or the genetic correlations between traits without controlling them. Indeed, even if laboratory studies are required for such controls and for insight in molecular pathways and are logistically easier, they often bias heritability measures because of artificial and novel environment; moreover, laboratory stocks are rather inbred [4]. Therefore and thanks to the new tools, the need for more studies of MEs under natural conditions has brought a new literacy on MEs in the wild. To my knowledge, no study to date has focused on MEs in the wild. Here, I review the main results made in recent years in this evolutionary ecology domain concerning MEs on life history traits and evolution at individual and population scale.
Maternal effects at an individual scale
Prenatal maternal effects
Prenatal in utero or in ovo development is one of the most important time in an animal's life. Current physiological status of the mother, maternal life history and birth/nest/oviposition environment choice are the main prenatal MEs.
The physiological status of the mother impacts on various aspects of the OP on short and long-term scales. The immune status of the mother during the prenatal period is of highest importance for the health of the offspring. Mammal mothers can infect their offspring through the placenta. In apes, studies show the transmission of the simian immunodeficiency virus from mother to offspring [5]. The use of non-invasive methods of fecal samples analysis established the link between individual infection and relatedness of infected monkeys. Infection is not the only immune ME that has been reported in the wild. In a recent study [6], Garnier et al. vaccinated three marine birds populations against Newcastel disease. An intergenerational transfer of specific antibodies occurred and protected the nestlings on a 20 days period. This ME has important positive consequences on the fitness of both mother and offspring.
The maternal stress status will also modulate the offspring development [7]. Here, MEs are due to placental hormone transfer. Glucocorticoids are the steroid hormones of stress for mammals. Their fecal quantification in a wild yellow-bellied marmot population reflected the level of stress due to predator pressure [7]. Older stressed mothers had smaller and female-biased litters and their sons were more likely to disperse, whereas younger unexperienced mothers displayed the opposite pattern: age-related MEs are correlated with an increase in maternal fitness.
Maternal life history also provides prenatal MEs. Among these MEs are age-related experience and senescence very important; social rank and previous gestations also play a role.
Maternal age is an easy parameter to survey in the wild and has been shown to be of great importance for OP [8]. According to the terminal investment hypothesis, older mothers should invest more in reproduction because their reduced remaining life diminishes their future reproductive potential. However, an other hypothesis assumes a decrease in reproductive success due to restrictive senescence [9]. In mammalian altricial species such as European rabbits, differences on pups body mass are due to maternal age [9]. Pups of medium-aged females had the highest growth rate, an effect which has been noticed in many small mammals species, since 1-year old mothers are often not fully-grown and might less efficiently conserve their energy because of lower body mass, and senescence effects reduce lactational performance in older 5-years old mothers. Noteworthy, OP is more affected by the present state of the female than by her past: in mountain goats Oreamnos americanus , former weaning success neither influence current weaning success nor kid sex [10]. Hence, it is the current maternal state that really influences its OP.
Mothers are from far the main link between an embryo and its future environment. However, the embryonic contemporary environment is made of its siblings. That is why parameters such as the brood sex-ratio or the numbers of siblings are important modulators of OP directly under maternal influence. Most mammal species bear more than one fetus per litter. Thus, in mixed-sex litters, female fetuses may be subjected to testosterone diffusion which affects their short and long-term phenotypes. Monclùs and Blumstein found that the proportion of male siblings in the litter explained 22 % of the variance in female anogenital distance, a common indicator of testosterone exposition, in wild yellow-bellied marmots [11]. These masculinized females had fewer survival rate, dispersed more and had decreased fecundity compared to females from female-biased litters. On a long-term scale, females with longer anogenital distance had lower lifetime reproductive success. Humans opposite sex twins also display minor hormonal MEs [12]••, with brother displaying reduced right jaw teeth asymmetry -a common male sexual dimorphism- and sisters showing deeper sensation seeking. However, cultural, social and statistical factors can moderate these conclusions.
The effects of time and place of parturition or oviposition on OP are mediated by maternal choices. In many insect species, the eggs develop on the resource patch they have been laid on. When possible, mothers choose the best food quality on which to lay eggs (but see box 2). In the seed beetle Stator limbatus , Fox and Mousseau [13] observed no difference on progeny development and survival rate when larvae were laid on high-quality host plant Acacia greggii . Nonetheless, on poor-quality host plant Cercidium floridum , larval performance was lower because of smaller eggs. On these low-quality plants, larvae have to burrow through the coat of the seed to enter it. However, the coat contains noxious allochemicals. The authors suggest that the exposition to such chemistry would be shorter for bigger larvae that dig faster. The MEs would here come from oviposition choice affecting larval survival rate. The choice of oviposition, nestling or burrowing site can also induce MEs because of localized physical and/or chemical parameters detected by the mother. Morrongiello et al. [14] reported a reduction in egg size and a rise in the egg number of the freshwater fish Nannoperca australis when the environment quality declined and its variability increased. They argue that random mortality due to highly transitory drought would nullify the benefits of having large offspring. Because maternal fitness is independent of offspring egg-size, increasing fecundity will allow more eggs to spread throughout the river before it stops flowing. Thus, eggs will disperse as far as in permanent water where they will be more likely to survive. Predator sensing accounts too: Cope's gray treefrog Hyla chrysoscelis mothers preferentially deposit eggs in ponds that do not contain predators or intra-specific competitors chemicals [15].
MEs can also come from birth site choice. Indeed, it is very important for females, when they can, to regulate their offspring birth date in order to fit the best environmental conditions. Wilson et al. found that Soay sheep ( Ovis aries ) birth date was affected for 19.7% by maternal genes, more than the individual genes [16]•. As birth date affects birth weight and as both parameters are under natural selection, MEs of birth timing will act upon OP.
Early MEs can result in adaptive phenotype to enhance offspring quality. However, evolutionary ecologists must not neglect postnatal MEs in their studies. An example is the burying beetle Nicrophorus vespilloides : young reproducing females had shorter eggs than females who delayed reproduction a few weeks after sexual maturation [17]. Young females displayed increased parental care in front of enhanced offspring begging. This adjustment is not grounded on previous learning, which shows a covariation in prenatal and postnatal MEs. Hence, it is also fundamental to consider postnatal MEs and their relationships with prenatal MEs.
Postnatal maternal effects
Supplying food, repelling predators, … MEs can participate at ensuring the vital needs of altricial young animals through non molecular nor cellular effects such as behavior or hormones.
Newly born brood first need to benefit from available and high-quality food. Cam et al. [18] associated the length of the rearing period of the seabird kittiwake Rissa tridactyla with a long-term augmentation of reproductive performance. Longer rearing period improves both amount of resources and survival rate during the first winter at sea. Because the rearing period depends on maternal choices, MEs are involved in long-term enhancement of OP. Groothuis et al. proposed [19] an explanation for food allocation by examining the effects of prenatal exposure to maternal androgens. Hormone deposition in the egg enhances the begging frequency in zebra finches; as this could stimulate the motor system development and increase hunger motivation, it seems logical that hormonal MEs will improve chick phenotype through prolonged care and increased food provisioning. Noteworthy, bird males also display altricial behavior resulting in paternal effects.
The nutritional supply is at first sight threatened by numerous siblings with whom to share parental food. However, having siblings gives more chance to guarantee the vital thermal need. In European rabbits [20]•, this positively affects biomass conversion of maternal milk by decreasing thermal need. A 3 pups litter size is the best trade-off between food sharing and thermal benefits under cold conditions. MEs positive or negative impacts are thus submitted to environmental variations.
Another vital need is to avoid predators. Mothers commonly defend their progeny, as observed in European rabbits [21] by repelling predators; after emergence of the pups, during the risky nest period, lactating mothers stayed near the burrow where they displayed aggressive behavior against birds and other females. Indeed, on his 5-years study, Rödel pointed out a 7% higher infanticide rate in groups where the female hierarchical structure was heterogeneous and age-dependent. In same-age females group, social instability could increase infanticide level because of the competition for breeding burrows and the decrease of future density of pups. An interesting mechanistic approach of anti-predator response by Weaver et al. [22] brought out an epigenetic process. Increased pup licking and grooming and arched-back nursing changed the epigenetic status of a glucocorticoid receptor promoting gene, which in turn modified hypothalamic-pituitary-adrenal axis activity, involved in defensive responses. Therefore, this maternal predation perception could have been selected because it would be a forecasting cue of offspring environmental condition. Even if this work was led on laboratory rats, similar results are apparent in insects or reptiles [22].
Once the survival of the offspring is ensured, reproductive need occurs, which can also be affected by MEs. In a black-headed gull wild population, Eising et al. [23] manipulated yolk hormone levels and analyzed the effect on nuptial plumage when the chicks were 10 months of age. Maternal androgens in the egg have postnatal effects on the development of nuptial plumage and rate of sexual displays. This could be due to a rise in androgen sensitivity because of a receptor up‑regulation. Another explanation would consist in higher androgen production by early hormone exposure. Such MEs on reproductive phenotype have also been emphasized in other mammals species, including humans. Reproductive postnatal MEs also exist in other taxa such as insects. Mousseau and Dingle presented the influence of MEs on the production of sexual forms [24]. The production of both male and oviparae in Megoura viciae (gynoparae in Aphis fabae and Myzus persicae ) are under the endocrine control of the mother. Sexual forms appear when females are exposed to short-day photoperiods, which is thought to allow to fit with environmental conditions.
Maternal effects at a population scale
Since MEs have an influence on individual lifespan and reproductive life history traits, they will change population dynamics and evolution.
Maternal effects influence the dynamic of populations
As previously exposed, MEs can be important for offspring survival [21], which constitutes a main population dynamic factor. MEs also affect dispersal behaviors. Duckworth [25] identified in a wild population of western bluebirds ( Siala mexicana ) the factors leading to the production of aggressive dispersive males instead of non‑aggressive philopatric males. The dynamics of range expansion depends on the availability of nest cavities. When the competition for this resource is high, females deposit more androgen in the eggs, triggering earlier production of more sons. These aggressive sons are less likely to cooperate with their pairs and tend to disperse in new territories where their conspecifics density will be low. Similar results have been found in rodents [12]••. Thus, MEs deeply influence the range expansion of this species. Crowding can result in low food-availability which itself affects species dynamics through MEs. In marine invertebrate species Alderia modesta [26], larvae can have early spontaneous metamorphose in the egg mass or delay the process until they encounter their obligate adult food alga. When parents were starved, clutches contained lower level of spontaneous metamorphosed larvae, enhancing the potential dispersion of larvae. As MEs are widespread, it is required to take them into account for the modeling of population dynamics, especially population size. Benton et al. [27] claimed that MEs are to be considered for understanding the gap between environmental cues and population response.
How do maternal effects affect the species evolution?
In a major paper, Wolf et al. [28] predicted that the lack of direct genetic variation for MEs-dependent traits does not hamper their evolution since these traits encounter heritable environmental variation due to MEs. MEs would thus prevent or favor response to selection. Intergenerational selection on these traits will lead to evolution: phenotypic response to selection in the previous generation (mothers) impacts on the current generation (offspring) phenotypic response. The process never ends but sharply decreases after the first generation following the end of selection [28]. In order to test Wolf's assumption, heritability measures – heritability accounting for how much of a trait variation proceeds from variation in genetic factor– were undertaken in the field. Indeed, Fisher's theorem of natural selection links traits closely associated with fitness with a decrease in heritability compared to traits not tightly related to fitness. In a wild population of red deer ( Cervus elaphus ) [4], MEs were prevalent to explain life history traits such as birth weight, longevity or female adult breeding success. Omitting this MEs on phenotypic variance would have overestimated their heritability. This omission could have been a frequent mistake in former evolutionary studies which now deserve to be avoided (see figure 1). For instance, heritability changes due to MEs can be up to five times greater than what was predicted when not considering them in a red squirrel wild population [29]. This study also highlights the intergenerational link due to selection: the stronger the selection was on growth rate in previous generations (grandparental and parental), the more the current juveniles respond to it, clearly indicating a microevolutionary process of evolutionary momentum. Therefore, MEs are of great importance in evolutionary dynamics works which now have to include them to understand the contributions from previous generations.
Figure 2. MEs can entail apparent population or evolutionary dynamics.
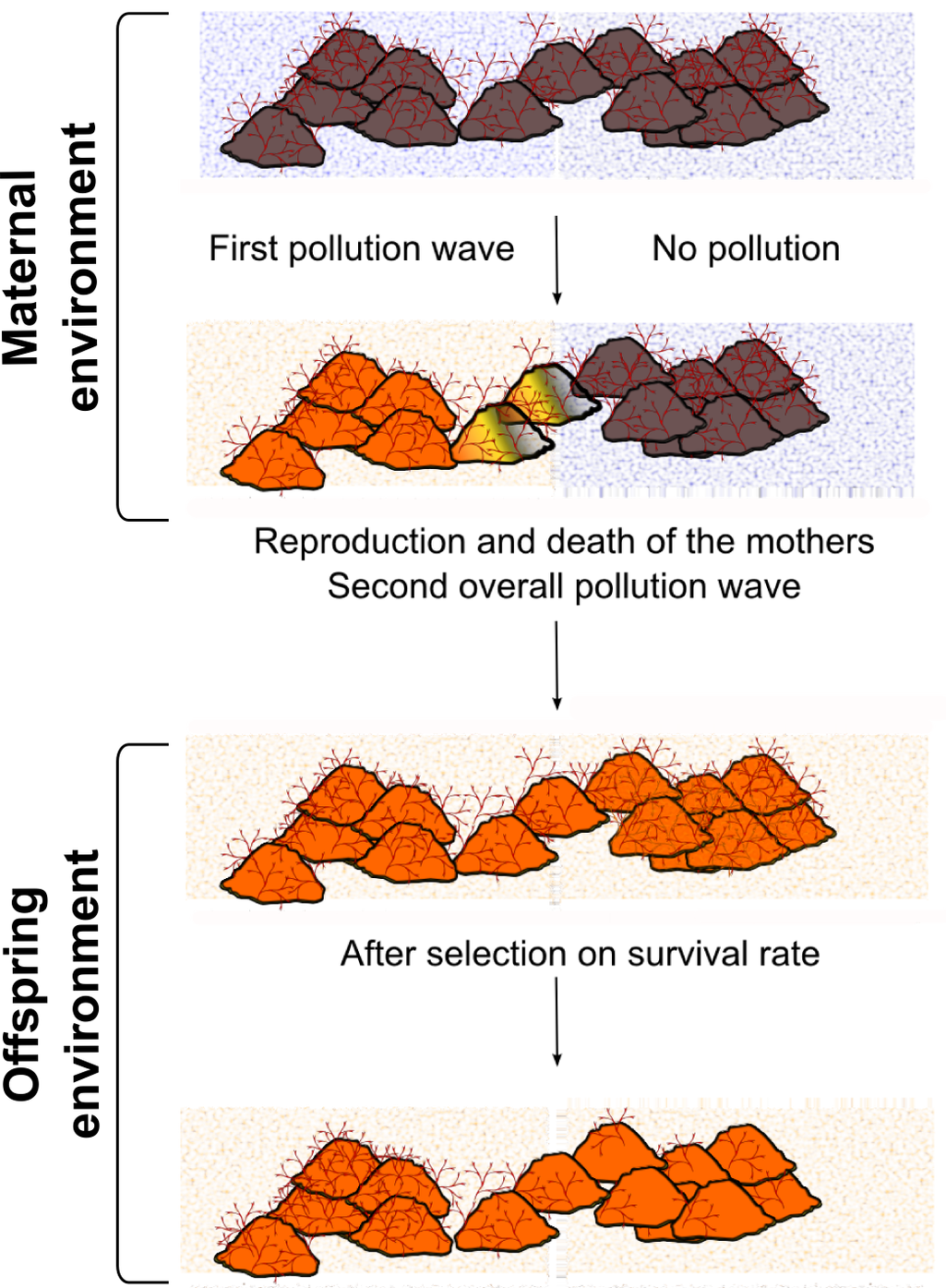
Bugula neritina is a colonial marine static invertebrate often settling on rocks in subtropical to temperate zones and sensitive to copper common pollution [36]. A) After a first pollution wave partially affecting the habitat (e.g. because of marine streams), half of the mothers encountered life in the polluted zone. These mothers produced larvae that were more resistant to copper pollution than larvae produced by non-exposed mothers. B) If a second pollution wave occurs in the whole habitat zone during the next year (so that mothers will have disappeared), there will be more B. neritina coming from exposed mothers than larvae whose mothers were non-exposed. Mechanistically, it is likely (but unproved) that as in other marine species [37], maternal deposition of metalloprotein mRNA reduces the noxious effects of copper pollution. Thus, if the first pollution wave was not well documented or the MEs well known, it will result in misinterpretation of population dynamics. The observed reduced global heterozygosity (Wahlund effect) does not result from a bottleneck due to genetic selection. Hence, if one is searching for a specific locus to explain the adaptation to pollution (e.g. through GWAS) or for evidences of a colonization phase, no result will outcome.
Conclusion
MEs are capital to fully understand the consequences and evolution of maternal influence on the life history traits of their offspring in wild populations [1]••[2]. MEs operate pre- and postnatally and can modulate the response to selection in animal species [28].
Since MEs can be passive or active [1]••, further studies are required to elucidate their physiological mechanisms in order to understand both their function and genetic bases. Recent advances in numerous taxa have underlined the interspecific similarities of MEs, e.g. the role of maternal androgens [23]. However, generalizations are not to be made because MEs deeply rely on genetic and environment interactions. Because they act on the raw material for selection, MEs can change evolutionary processes [16]•. Focus needs to be made for the evolutionary importance of MEs in humans, for instance to explain the intrauterine effects in twins, or the development of cultural behavior. Modeling has been built to understand how MEs participated in the evolution of human life history traits [30] (highly mother-dependent babies, short reproductive period with reproductive senescence and menopause, extended post-reproductive lifetime), emphasizing the role of maternal care and grand‑maternal care, which consists in helping their daughter – this being a long-term MEs indirectly increasing their own fitness. Such models, which are corroborated by non-human primates observations [31], now deserve verification « in the field ».
In future studies, MEs comprehension will improve conservation and reintroduction programs. Since they can buffer offspring from environmental change by acting upon them, MEs result in rapid trans-generational plasticity. Climate change has already been proved to shape Columbian ground squirrels date of emergence from hibernation through genetic selection [32], but it seems likely that MEs will play a major (and faster) role in animal species response to global warming in the coming years.
Acknowledgments
I thank B. Laperrousaz for helpful discussions, A. Corbin for formal advice and M. Bossennec, M. Calvez and F. Figon for their precious remarks.
References and recommended reading
Papers of particular interest have been highlighted as:
● of special interest
●● of outstanding interest
1. J.
Marshall D, Uller T:
When is a
maternal effect adaptive?
Oikos
2007,
116
:1957–1963. [
Full Paper
]
●● Main reflexion on the potential
individual and evolutive consequences of MEs on the fitness.
2.
Mousseau TA, Fox CW:
The
adaptive significance of maternal effects
.
Trends in
Ecology & Evolution
1998,
13
:403–407. [
Full Paper
]
●● A major paper which highlighted
the roles of MEs in evolutionary ecology.
3.
Wilson AJ, Réale D, Clements MN, Morrissey MM, Postma E, Walling CA,
Kruuk LEB, Nussey DH:
An
ecologist's guide to the animal model
.
Journal of
Animal Ecology
2010,
79
:13–26. [
Full Paper
]
● One of the clearest insight into
the statistical tools used to study MEs.
4. Kruuk LEB, Clutton-Brock TH, Slate J, Pemberton JM, Brotherstone S, Guinness FE: Heritability of fitness in a wild mammal population . Proceedings of the National Academy of Sciences 2000, 97 :698–703.
5. Rudicell RS, Piel AK, Stewart F, Moore DL, Learn GH, Li Y, Takehisa J, Pintea L, Shaw GM, Moore J, et al.: High prevalence of Simian Immunodeficiency Virus infection in a community of savanna chimpanzees . J. Virol. 2011, 85 :9918–9928. [ Full Paper ]
6. Garnier R, Boulinier T, Gandon S: Coevolution between maternal transfer of immunity and other resistance strategies against pathogens . Evolution 2012, 66 :3067–3078. [ Full Paper ]
7. Monclús R, Tiulim J, Blumstein DT: Older mothers follow conservative strategies under predator pressure: The adaptive role of maternal glucocorticoids in yellow-bellied marmots . Hormones and Behavior 2011, 60 :660–665. [ Full Paper ]
8. Rödel HG, Von Holst D, Kraus C: Family legacies: short- and long-term fitness consequences of early-life conditions in female European rabbits . Journal of Animal Ecology 2009, 78 :789–797. [ Full Paper ]
9. Rödel HG, Prager G, Stefanski V, Von Holst D, Hudson R: Separating maternal and litter-size effects on early postnatal growth in two species of altricial small mammals . Physiology & Behavior 2008, 93 :826–834. [ Full Paper ]
10. Côté SD, Festa-Bianchet M: Offspring sex ratio in relation to maternal age and social rank in mountain goats ( Oreamnos americanus ) . Behav Ecol Sociobiol 2001, 49 :260–265. [ Full Paper ]
11. Monclús R, Blumstein DT: Litter sex composition affects life-history traits in yellow-bellied marmots . Journal of Animal Ecology 2012, 81 :80–86. [ Full Paper ]
12.
Ryan BC, Vandenbergh JG:
Intrauterine position effects
.
Neuroscience & Biobehavioral
Reviews
2002,
26
:665–678. [
Full Paper
]
● This study compares a broad range
of taxa allowing better reflexion.
13. Fox CW, Mousseau TA: Larval host plant affects fitness consequences of egg size variation in the seed beetle Stator limbatus . Oecologia 1996, 107 :541–548. [ Full Paper ]
14. Morrongiello JR, Bond NR, Crook DA, Wong BBM: Spatial variation in egg size and egg number reflects trade-offs and bet-hedging in a freshwater fish . Journal of Animal Ecology 2012, 81 :806–817. [ Full Paper ]
15. Resetarits WJ: Oviposition site choice and life history evolution . Amer. Zool. 1996, 36 :205–215. [ Full Paper ]
16.
Wilson AJ, Coltman DW, Pemberton JM, Overall ADJ, Byrne KA, Kruuk
LEB:
Maternal genetic effects set
the potential for evolution in a free-living vertebrate
population
.
Journal of Evolutionary Biology
2005,
18
:405–414. [
Full Paper
]
● A clear example of the importance
of long-term study in the field.
17. Lock JE, Smiseth PT, Moore PJ, Moore AJ: Coadaptation of prenatal and postnatal maternal effects. The American Naturalist 2007, 170 :709–718. [ArticleType: research-article / Full publication date: November 2007 / Copyright © 2007 The University of Chicago]
18. Cam E, Monnat J-Y, Hines JE: Long-term fitness consequences of early conditions in the kittiwake . Journal of Animal Ecology 2003, 72 :411–424. [ Full Paper ]
19. Groothuis TGG, Müller W, Von Engelhardt N, Carere C, Eising C: Maternal hormones as a tool to adjust offspring phenotype in avian species . Neuroscience & Biobehavioral Reviews 2005, 29 :329–352. [ Full Paper ]
20.
Rödel HG, Hudson R, Holst D:
Optimal litter size for individual growth of European
rabbit pups depends on their thermal environment
.
Oecologia
2008,
155
:677–689. [
Full Paper
]
● An ingenious way
to trade between field observation and experimental
interventions.
21. Rödel HG, Starkloff A, Bautista A, Friedrich A-C, Von Holst D: Infanticide and maternal offspring defence in european rabbits under natural breeding conditions . Ethology 2008, 114 :22–31. [ Full Paper ]
22. Weaver ICG, Cervoni N, Champagne FA, D'Alessio AC, Sharma S, Seckl JR, Dymov S, Szyf M, Meaney MJ: Epigenetic programming by maternal behavior . Nature Neuroscience 2004, 7 :847–854. [ Full Paper ]
23. Eising CM, Muller W, Groothuis TG.: Avian mothers create different phenotypes by hormone deposition in their eggs . Biology Letters 2006, 2 :20–22. [ Full Paper ]
24. Mousseau TA, Dingle H: Maternal effects in insect life histories . Annual Review of Entomology 1991, 36 :511–534. [ Full Paper ]
25. Duckworth RA: Maternal effects and range expansion: a key factor in a dynamic process? Phil. Trans. R. Soc. B 2009, 364 :1075–1086. [ Full Paper ]
26. Krug PJ: Bet-hedging dispersal strategy of a specialist marine herbivore: a settlement dimorphism among sibling larvae of Alderia modesta . Mar Ecol Prog Ser 2001, 213 :177–192. [ Full Paper ]
27. Benton TG, Ranta E, Kaitala V, Beckerman AP: Maternal effects and the stability of population dynamics in noisy environments . Journal of Animal Ecology 2001, 70 :590–599. [ Full Paper ]
28. Wolf JB, Brodie III ED, Cheverud JM, Moore AJ, Wade MJ: Evolutionary consequences of indirect genetic effects . Trends in Ecology & Evolution 1998, 13 :64–69. [ Full Paper ]
29. McAdam AG, Boutin S: Maternal effects and the response to selection in red squirrels . Proceedings of the Royal Society B: Biological Sciences 2004, 271 :75–79. [ Full Paper ]
30. Pavard S, Branger F: Effect of maternal and grandmaternal care on population dynamics and human life-history evolution: A matrix projection model . Theoretical Population Biology 2012, 82 :364–376. [ Full Paper ]
31. Maestripieri D, Lindell SG, Higley JD: Intergenerational transmission of maternal behavior in rhesus macaques and its underlying mechanisms . Developmental Psychobiology 2007, 49 :165–171. [ Full Paper ]
32. Lane JE, Kruuk LEB, Charmantier A, Murie JO, Coltman DW, Buoro M, Raveh S, Dobson FS: A quantitative genetic analysis of hibernation emergence date in a wild population of Columbian ground squirrels . Journal of Evolutionary Biology 2011, 24 :1949–1959. [ Full Paper ]
33.
Wolf JB, Wade MJ:
What
are maternal effects (and what are they not)?
Philosophical Transactions of the Royal Society B: Biological
Sciences
2009,
364
:1107–1115. [
Full Paper
]
●● The first theoretical work to
read before starting the study of MEs.
34. Martin JGA, Festa-Bianchet M: Age-independent and age-dependent decreases in reproduction of females . Ecology Letters 2011, 14 :576–581. [ Full Paper ]
35. Mayhew PJ: Herbivore host choice and optimal bad motherhood . Trends in Ecology & Evolution 2001, 16 :165–167. [ Full Paper ]
36. Marshall DJ: Transgenerational plasticity in the sea: context-dependent maternal effects across the life history . Ecology 2008, 89 :418–427.
37. Lin H: Maternal transfer of cadmium tolerance in larval Oreochromis mossambicus . Journal of Fish Biology 2000, 57 :239–249. [ Full Paper ]