The story of symbiosis with zooxanthellae, or how they enable their host to thrive in a nutrient poor environment.
21 - 01 - 2013
Abstract
The high number of marine invertebrate host that have evolved towards establishing symbiotic relationships with the zooxanthellae (genus Symbiodinium ) suggests the highly valuable competitive advantage provided by such associations. Relationships may be either very specific or generalist between the numerous clades of algae and their hosts. This review examines the genetic diversity of zooxanthellae in relation to that of their hosts, and studies the nature and the roles of this intimate interaction, in an attempt to identify the traits that have resulted in their extraordinary evolutionary success. Despite their great competitiveness, such associations remain sensitive to environmental perturbation and are increasingly threatened by bleaching events. Studying their resilience and their ability to adapt to rapid environmental change is one of our greatest actual challenges in marine biology.
Table of Contents
Introduction
According to de Bary's original definition, a symbiosis is a long-term relationship between two different species. In this review, we focus on those involving zooxanthellae, which is the common term used to refer to unicellular dinoflagellate photosynthetic algae of the genus Symbiodinium (family Symbiodiniaceae, order Suessiales, class Dinophyceae). Over the past 25 years, the use of new molecular tools has led to the identification of nine major clades (A to I) [1–3], with each clade being characterized by a considerable level of diversity [4]. Symbiodinium can live freely in sea water or be associated with a great variety of hosts, ranging from Foraminifera to marine invertebrates, including Cnidaria (corals (Figure 1), jellyfish, ane-mones, zoanthids), Mollusca (snails and clams), Platyhelminthes (flatworms) and Porifera (sponges). From one generation to another the symbiont may be transmitted either directly from the parent to the larvae, or indirectly, implying that the host must acquire them from the surrounding environment [5–7].
Figure 1.
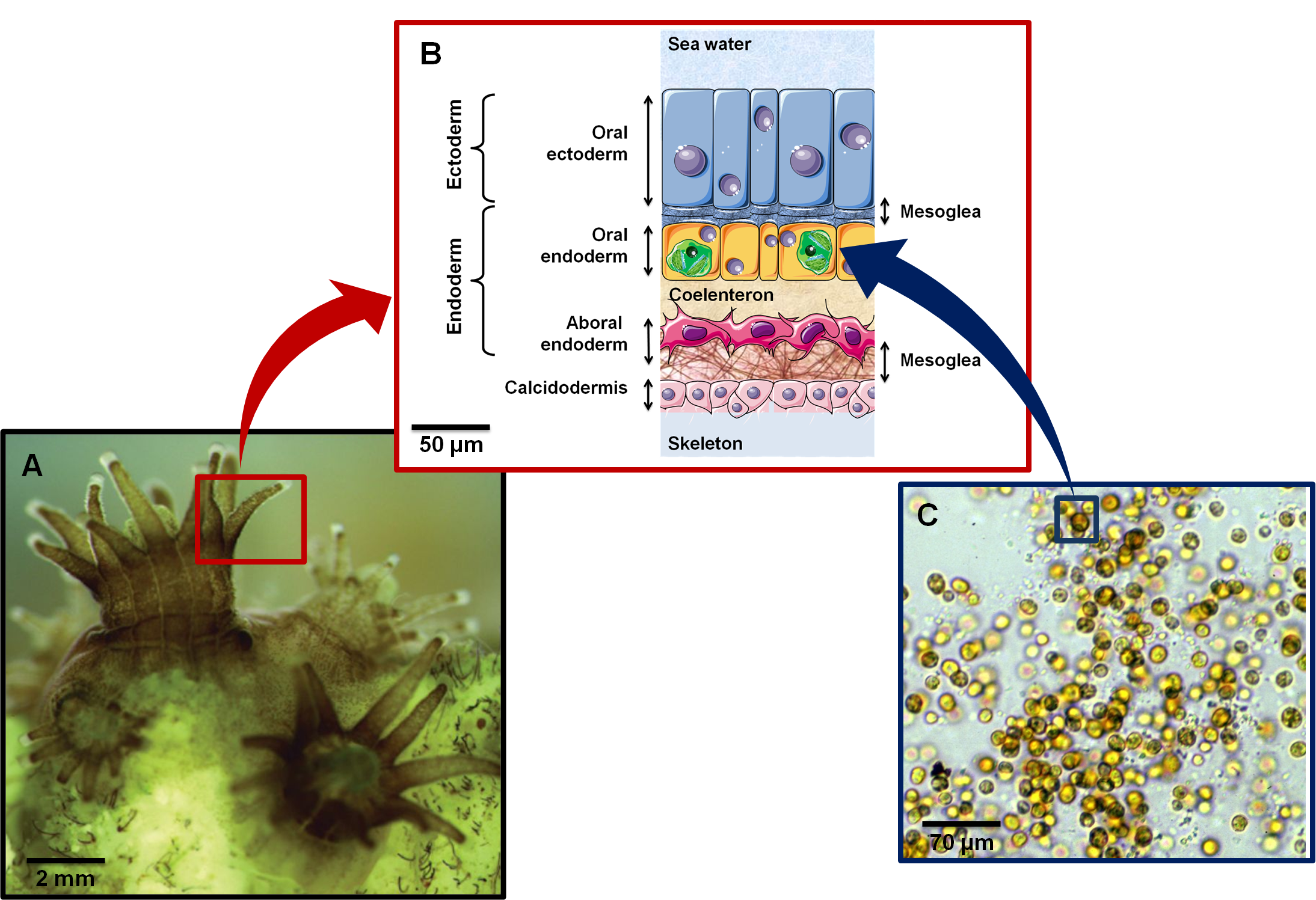
(A) Close up of coral polyps (Image credit: Alison Moulding / National Coral Reef Institute). (B) Cross section showing histological organization of a symbiotic and calcifying Anthozoan. Anthozoa are composed of two cell layers: ectoderm (epidermis) and endoderm (gastrodermis). Symbiodinium (represented as green structures in oral endoderm) are found in peri-vacuolar host membranes (symbiosome) in the gastrodermal cells. (C) Symbiodinium kawagutii under a light microscope. Yellow-brown color is due to characteristic dinoflagellate pigments (diadinoxanthin, peridinin) as well as chlorophylls a and b (Image credit: Scott R. Santos, Department of Biological Sciences, Auburn University).
As such, we question here the degree of specificity/flexibility of the relationship between the host and its symbionts: which molecular mechanisms permit algal-host recognition and fidelity [8]•,[9]• ? What evolu-tionary advantages are provided by stable or on the contrary flexible relationships [11]? Which factors modulate this degree of specificity [12],[13]••? Those associations gives rise to an intense nutrient recycling between the partners, with a bilateral exchange of metabolites [14,15],[16]•, as well as the synthesis of novel metabolites, not produced by either organism independently. We will consider the extend to which the interaction modifies their gene expression [17], metabolic profile [18,19] and anatomy, in order to unveil how those changes may maximize the life history strategy of the symbiosis [20,21]. Because such symbioses form the basis of the highly productive coral reefs ecosystems, and because they are extremely threatened by global warming, they have been intensely studied over the past two decades [22],[23]•. We finally concentrate, in this review, on the maintenance mechanisms of symbiotic associations and the reason for their collapse [24–26].
What is the extend of the diversity of symbiosis with Symbiodinium ?
Genetic diversity
Until the 1970s, although different in terms of behavior, physiology, ultrastructure and infectivity, all symbiotic dinoflagellates were all referred to as Symbiodinium [27]. It is only with the development of new molecular tools that the vast diversity of the genus Symbiodinium was revealed [10,28]; they originally consisted in the use of DNA hybridization, restriction fragment length polymorphisms and denaturating gradient gel electrophoresis. Later, the use of nuclear small subunit ribosomal DNA (18S-rDNA), direct sequencing and real time PCR led to the same conclusion that diversity within the genus was unexpectedly high, and eventually led to the creation of the still existing classification of Symbiodinium , composed of nine clades ranging from A to I (Figure 2). Clade A is the most ancestral clade; it appeared 65 to 50 Million years ago and all other clades have diverged from it. During Miocene, many radiations took place within every clade [13]••,[29]. Clade I was isolated very recently from soritid foraminifera [30]•• (Figure 2).
The study of the chloroplastic and mitochondrial genes have further confirmed this phylogeny, but have also recognized that each clade is itself composed of numerous strains [2]. Intra-cladal diversity was then estimated using gene sequences of the ribosomal operon (18S, 5.8S and 28S rDNA) and ribosomal internal transcribed spacer sequences (ITS-1 and 2) [1,13,28], proposing that at least 160 distinct Symbiodinium types exist. Using additional loci to reach an even fineer-scale characterization of genetic diversity within each clade will facilitate the association of precise markers to specific strains and will eventually enable us to deal more accurately with the questions of host specificity and coevolution [3].
The host is a major evolutionary force in the maintenance of the genetic diversity; each host's cell offers a unique biochemical, morphological and structural environment, creating a distinct niche from any other cells. Indeed, an additional level of diversity in dinoflagellates can be appreciated by the large number of taxa that form symbiotic interactions with them (Figure 2). The hosts include Foraminifera and marine invertebrates from fous phyla: Cnidaria (corals, jellyfish, anemones, zoanthids), Mollusca (snails and clams), Platyhelminthes (flatworms) and Porifera (sponges).
Molecular markers are now used to try and correlate Symbiodinium phylogeny with their adaptive strategy, such as their geographical repartition, thermal resistance and host transmission strategy [2].
Figure 2. Phylogenetic relationships between the major clades of Symbiodinium.
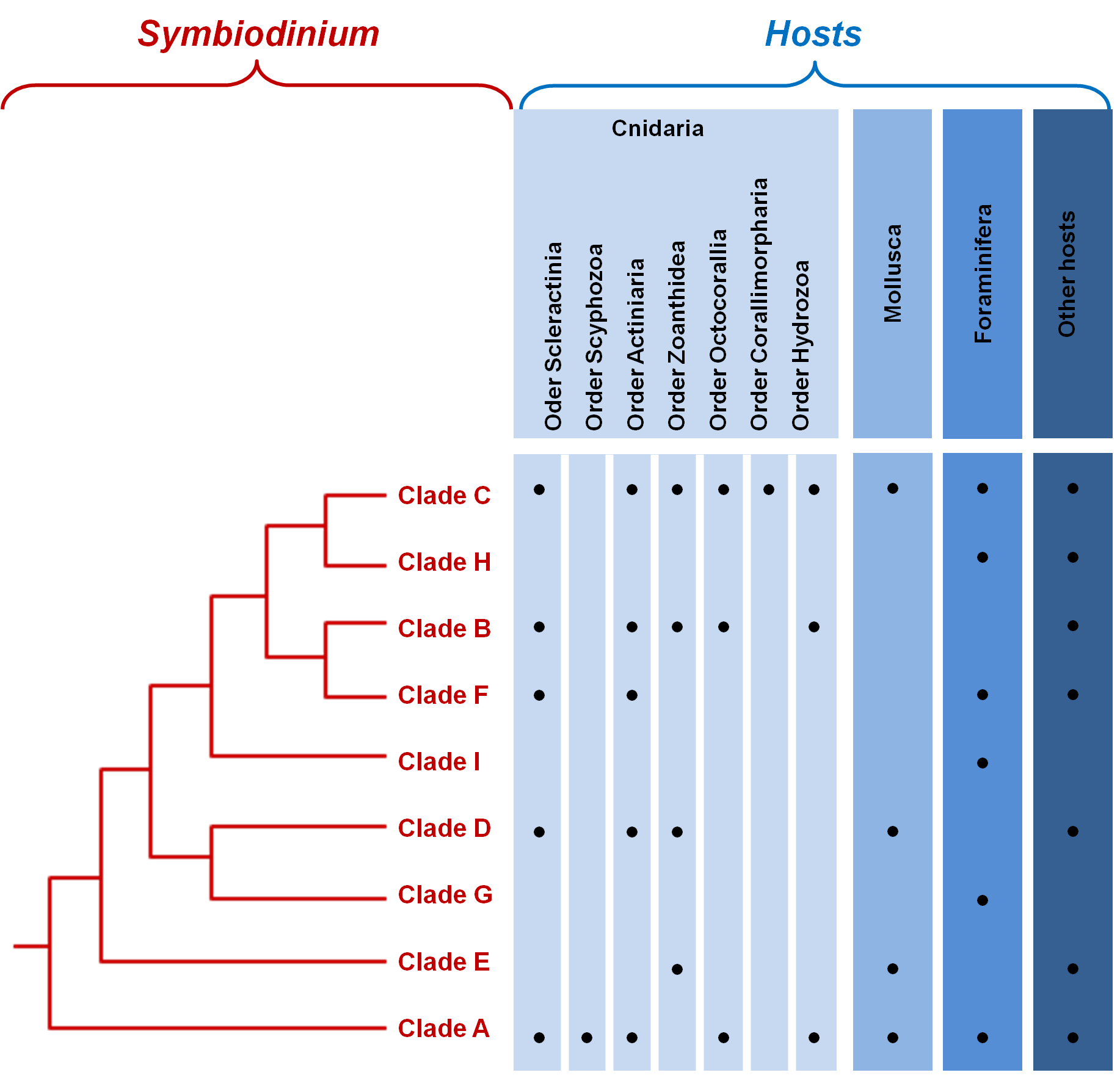
Taxa within each clade of Symbiodinium exhibit high genetic diversity.
Diversity of relationships; from specificity to flexibility
Symbiodinium types have been identified to establish non-random relationships with host species, nonetheless, specificity and fidelity of relationship over space and time may be modulated by external conditions [21,31,32]; indeed, each host, in a given environment, only presents a subset of the algae it could potentially arbor in another habitat [10].
Specificity of the relationship
Symbiodinium exhibiting a high degree of specificity for their host are refered to as “ecological specialists”. Such fidelity is illustrated by some foraminifera, that form associations with Symbiodinium type F only, or giant sea anemone which occurs in symbiosis with clade A and B only [34]. Although hosts larvae may obtain their symbionts from sea water, meaning that infections by all symbionts present in the environment are possible, host-algal specificity is then usually re-established in the adult phase. The selective pressures, particularly in highly fluctuating temperate environments, seem to be a reason for specific host-algae relationship [13]. The question of co-evolution, that is correlation between the evolutionary history of Symbiodinium and their host's needs to be addressed using fine molecular markers [1,13]. A lack of co-evolution may imply that flexible relationships are evolutionarily more valuable than specific associations. To what extend then can depth, light intensity, temperature, latitude and longitude turn “specialists” into “generalist symbionts”?
Flexibility of the relationship
Some species can host a number of symbiont types [12] and a single symbiont type can be found within multiple hosts. Depth/irradiance gradients have been shown to influence symbiont repartition for example, Zoanthus sansibaricus can associate with different Symbiodinium types according to depth [31], reflecting the “sun-loving” or “shade-adapted” nature of the algae [13]. Therefore, not only does host specificity determines Symbiodinium niche, but also external parameters such as light intensity, temperature and turbidity [12,32], and this reduction in host-algae fidelity is highly valuable in locally changing environments.
At a bigger scale, variation in host-symbiont pairing with latitude has also been considered; in Australia, the same host species contains Symbiodinium type C in the northeastern tropical waters and type B in the South Eastern [4]. Functional diversity of genetically varying strains of algae partly explains why flexible association with their host are valuable; each may bring different benefits to their host, enabling its adaptation to distinct environments.
What kind of interactions take place between the partners?
A symbiosis is not a mere addition of two partners, rather, it gives rise to new metabolic profiles and increased competitiveness. Therefore, the partners should not be studied separately. Intact symbiosis should be used for the study of metabolomics.
Metabolic interactions
Metabolic interactions consist in a system of nutrient recycling, between the host and its symbionts, providing them with a competitive advantage in the shallow and low in nutrients tropical waters [14]. The autotrophic algae acquires nutrients ans metabolic breakdowns from the host (Figure 3), and produces organic compounds to fulfill not only the symbionts' requirements for growth and respiration but also up to 95% of the host's energy demand. In corals, these exchanges of nutrients must occur through the symbiosome membrane, host derived membrane surrounding the symbionts, which allows the host to control the metabolite transfer to the algae (Figure 1). In tridacnid clams, the symbionts are not intracellular, rather, they occupy fine tubules originating from the digestive diverticulum of the stomach, and are irrigated by the host haemolymph through which they can export molecules to clam's tissues [14].
Carbon uptake and fixation
Anthozoa have undergone a number of physiological changes, such as in the localization and activity of their enzymes, to actively transport Ci from the exterior to their internal endoderm and to perform CO2-concentrating mechanisms. These changes involve several proteins (carbonic anhydrase (CA), H+-ATPase) and allow dissolved inorganic carbon (DIC) to cross several membranes and reach sufficient concentration to be fixed by the Rubisco (ribulose-1,5-bisphosphate carboxylase / oxygenase) inside the algae (Figure 3).
The compounds produced by Symbiodinium are very diverse; glycerol is the major extracellular product, but other molecules include amino acids, glucose, maltose, Krebs cycle molecules, pyruvate, zooxanthellamine and zooxanthellatoxins [15]••. Exchanges between partners are not single way, instead, they consist of a genuine system of nutrient recycling as illustrated by lipid synthesis: Symbiodinium are the primary producers of corals' reserved energy and do it from host metabolism derived acetate [15]••.
In coral reefs, photosynthesis and calcification do not occur independently. The DIC necessary for photosynthesis is also needed to build the skeleton, composed of a mineral fraction (calcium carbonate (CaCO3) crystallized in aragonite) and an organic matrix (Figure 3). Light-Enhanced Calcification takes place in the presence of Symbiodinium ; (1) symbionts affect the DIC equilibrium, by taking up CO2 for photosynthesis, which directly favors the precipitation of CaCO3; (2) the algae remove phosphates that may inhibit calcification and thus enhance crystallization of CaCO3; (3) organic products of photosynthesis are precursor for organic matrix synthesis; and (4) energy and O2 supply to the host increases its metabolism and permit faster calcification [23]•.
Figure 3. Metabolic interactions and calcification process.
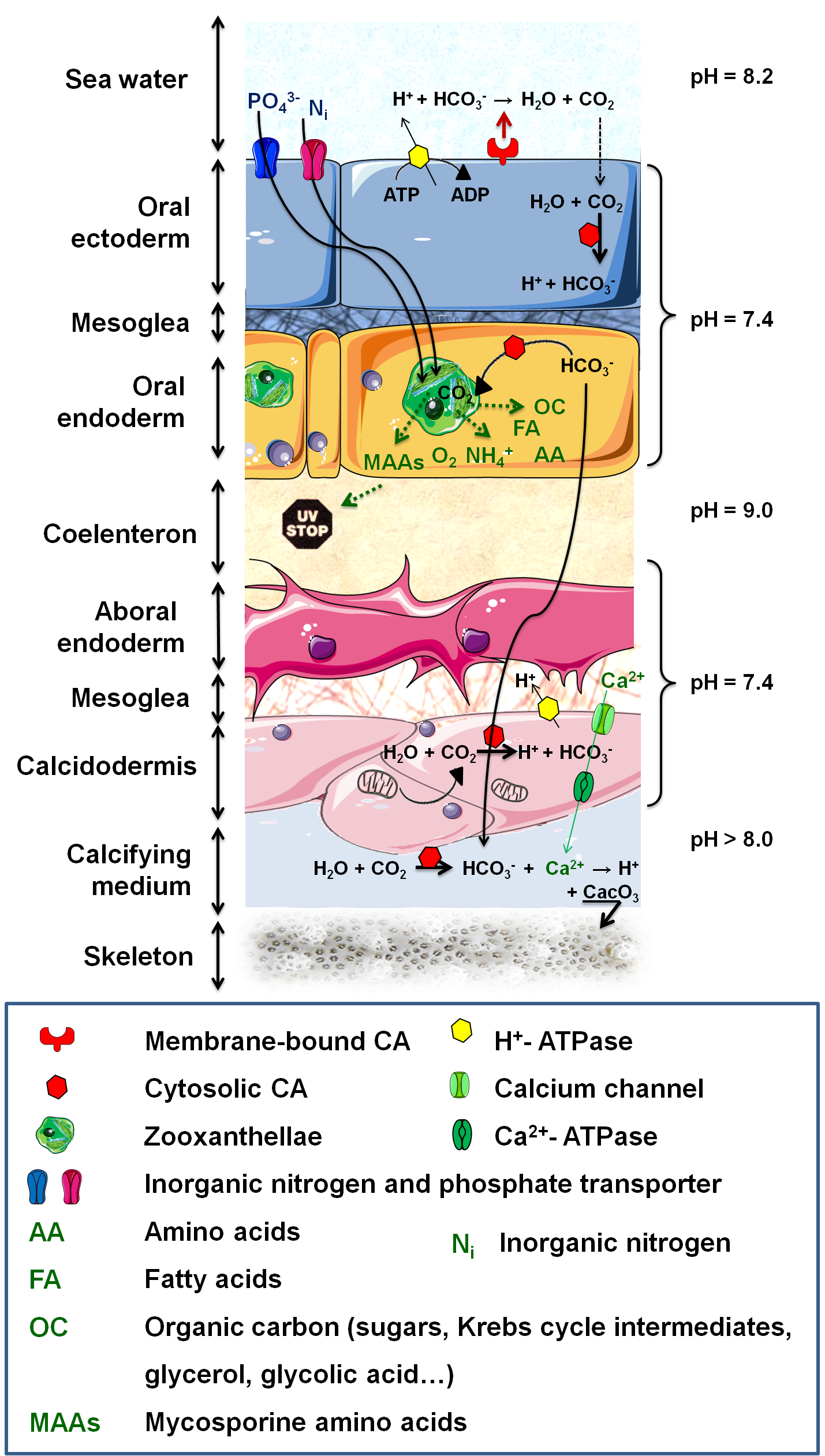
The autotrophic algae acquires metabolic breakdowns and nutrients from the host (inorganic carbon and nitrogen (NH4 +, NO3 -, N2), phosphate (PO4 3-) and other inorganic compounds). The algae produce enough organic molecules to fulfill up to 95% of the host's energy demand. Carbon uptake necessitates an H+-ATPase to acidify the boundary layer and lead to HCO3 - formation which is then quickly and externally dehydrated into CO2 by a membrane-bound CA (Carbonic anhydrase). CO2 subsequently diffuses into the host, where it is converted to HCO3 - by a cytosolic CA, preventing CO2 leakage. DIC (Dissolved inorganic carbon) is then supplied to (i) Rubisco for reduction or (ii) calcification sites. Biomineralization also necessitates a calcium (Ca) import from surrounding seawater and a protons export, resulting from mineralization process.
The global increase in CO2 concentration in the world's oceans leads to their acidification and to reduced calcification rates. Gene expression profiling may be an efficient tool in understanding the exact mechanisms of calcification and discovering whether calcifying organisms have sufficient physiological plasticity to adapt to a high-CO2 world [26].
Nitrogen uptake and fixation
Nitrogen is necessary for cell maintenance and for amino acid formation by the algae, and can be obtained in the form of ammonium (NH4 +) or nitrate (NO3 -).
NH4 + can be assimilated by both the algae and the host since they both possess the necessary enzymes, but it seems that the host is responsible for most of NH4 + assimilation and that it controls its availability for the algae, thus modulating the symbiont population. Indeed, nitrogen limitations slow down the passage from G1 to S phase in dinoflagellates, limiting growth, while keeping photosynthetic activity high [14]. Even so, nitrogen assimilated by the dinoflagellates can be transported back to the host in the form of “essential amino acids” that the host is unable to synthesize: histidine, isoleucine, leucine, lysine, phenylalanine, tyrosine and valine (Figure 3).
In contrast to NH4 +, NO3 - can only be assimilated by the symbionts, as only they possess the nitrate and nitrite reductases. This raises the question of the control of nitrogen release from the algae to the host and of the primary site for nitrogen assimilation in the symbiosis.
Ocean rising temperature and acidification negatively impact phosphate and nitrate uptake rates in corals [16]•, which adds to the growing body of evidence that corals will suffer from global change.
Phosphate uptake and fixation
Although phosphate is a limiting nutrient for algal growth, it has hardly received any attention in the context of invertebrate-algal symbiosis. We know that an elevated phosphate concentration increases coral growth, calcification rates and algal density [14]. We also know that the host can control Symbiodinium 's access to phosphate, since the later cannot diffuse across biological membranes at significant rates (Figure 3). But the exact mechanism for phosphate uptake is still unknown.
A modifying interaction for both partners
As a whole, the metabolic profiles, histological structures and enzymatic activities of both partners need to be modified and coordinated to ensure the best life history strategy.
Because of the presence of photoautotrophic symbionts within its tissues, the host has to adapt to significant diel variations in glucose concentration, pH and O2 concentration, ranging from 250% air saturation in the afternoon to less than 5% during night. An evolutionary adaptation of the host antioxidant defenses to high O2 production is the increased superoxide dismutase activity [18]. Symbiosis-induced modifications of the enzymatic profile of the host also include a 29 fold increase in CA activity in the presence of symbionts and the expression of specific membrane transporters necessary to provide the symbiont with numerous ions. It also seems that the symbiont is able to modulate host gene expression thanks to G-Proteins like receptors, distributed on the symbiosome membrane [7]. Marine invertebrates hosting Symbiodinium are forced to live in clear and shallow waters, where they are constantly threatened by high intensity solar radiations. To counter the effect of harmful light levels, their symbionts produce UV-absorbing "sunscreen" compounds, mycosporine amino acids (MAAs) (Figure 3). MAAs also have the potential to act as free radical scavengers, light-harvesting pigments, intracellular nitrogen reservoirs and osmotic regulators [19].
Hosts also display considerable morphological adaptation to symbiosis; tridacnid molluscs' body plan has undergone a 180° rotation and its digestive tract has been transformed to ensure maximal light exposition of the Symbiodinium . Even the Porifera and Cnidaria's body plans, well suited for light capture due to their large area/volume ratio, have evolved some morphological compromise to maximize light capture during the day and food capture at night.
The Symbiodinium are also drastically modified by the symbiosis; when free living, they alternate between motile and non-motile stages whereas in symbiosis, Symbiodinium are kept in a cell-dividing non-motile stage. The host-produced lectin SLL-2 can bind to specific glycoproteins on the algal cell surface and maintains the algae in this non motile stage [9]. The host can also produce host release factors to control the release of fixed carbon to its own tissues, by modulating Ca2+-calmodulin-mediated signaling in algal cell [35]. Another way in which hosts can control the algal density is by restricting nutrients availability [14]. At a greater temporal scale, corals also seem to reduce their symbiont population during cold season, when they perform heterotrophic feeding with host-derived organic substrates, and increase their population during the warm season [36]. Finally, density regulation can be achieved through expulsion or digestion of the algae. In turn, the algae enjoy many of the benefits of a macroscopic benthic lifestyle (maintenance in the photic zone, good water exchange) while being protected against herbivores.
How do host and symbionts communicate with each other?
The intimate cellular relationship taking place in symbiosis involves regulatory crosstalk between partners that allows the interaction to persist.
Starting the conversation between the partners; establishment of the symbiosis
Symbiodinium transmission corresponds to the starting of the conversation. Two different modes of transmission exist: horizontal (indirect, from the environment) and vertical (direct, from the parent). Mixed transmission also exists.
Indirect transmission is found in 85% of cnidarian species hosting Symbiodinium . Hosts must obtain them from seawater reservoirs [10] such as water column, benthos, and sediments [37]. Parrotfish guarantee the preservation of these reservoirs. Indeed, densities and diversity of viable algae are remarkable in their faeces; as such, they represent an efficient endozoochorous vector, contributing to the dispersal of Symbiodinium populations [38]. At each new generation, contact between the two partners at the right time and place is needed. Positive chemotaxis of motile Symbiodinium towards newly settled hosts increases the chances of contact between partners; they can adapt their swimming pattern, direction, and speed according to the host released chemical gradient [39]. Inter‑partner recognition occurs in the host-secreted mucus and is mediated by dinoflagellate glycans and host lectins [9]•. Then, once internalized by phagocytose, symbionts have to fight the host's immune defenses to avoid phagosome fusion with endolysosomes. Juveniles are associated with a greater variety of clades than adults [5,10, 37]. A “sorting” process occurs and leads to the specificity of adult hosts to a few dominant clades.
In vertically transmitted symbioses, there is no aposymbiotic phase and no need for symbiont selection or recognition molecules. Direct transmission has been observed more frequently in temperate algal-invertebrate symbioses, where exogenous sources of Symbiodinium are scarce, than in the tropical ones [7].
Regulatory crosstalk and persistence of the symbiosis
A constant molecular crosstalk involving molecular signals first and specific gene expression second maintains the interaction in a mutualistic state.
Changes in the host's immune response ensure its tolerance towards the algae. Those changes include the induction of TGFβ (transforming growth factor) in the host [8]•, or algae interference with the host vesicular recycling process, with RAB proteins, to avoid symbiosome fusion with lysosomes [7]. The new “arrested phagosome hypothesis” [40] shifts control of the symbiosis away from the host, and states that the release of algal products into the host can imitate the host endosomal digestive machinery and facilitate intra-host residence.
Expressed sequence tags are crucial in deciphering the molecular dialogue inferred by the symbiosis and identifying symbiosis induced genes [20], both in the algae, such as the H+- ATPase proton pump [41]• and in the host, such as the tissue specific CA isoforms genes, host gene sym32 (encoding a fasciclin domain protein), or pattern recognition receptors genes [17]. The level of expression of those “symbiosis genes” correlates with the quantity of algae in the host. It can be used as a biomarker for the host's health and for early detection of symbiosis breakdown. From a broader point of view, this conversation may well include more than two partners; cases of mixed infections are highly common, with 68% of corals bearing infections of more than one Symbiodinium clade [11]. This can be seen as a host bet-hedging strategy; maintaining a crosstalk with multiple taxa will empower it to benefit from various strains according to the conditions. This cosmopolitan conversation of the host with its numerous inhabitants increases its tolerance to variable environments; a considerable ecological advantage in times of rapid climate change.
Collapse of the relationship: bleaching events
Coral reefs are increasingly threatened by coral bleaching and mortality due to recent changes in salinity, high solar radiation, increased sedimentation or pollutants, elevated sea surface temperatures and global warming [22]. The diversity in symbiont harbored is one way of making them more tolerant in front of anthropogenic disturbances [42]. Indeed, bleaching has been proposed as an adaptative phenomenon, leading to the acquisition of new symbionts more appropriate to the new conditions. Corals with type C symbionts appear more likely to bleach than corals with type D symbionts, and increases in type D symbiont have been reported in coral reefs following bleaching events [24]. But still, little evidence exists that this “adaptative bleaching”, or shuffling for more thermally tolerant symbionts, is stable, neither that these corals will survive, nor that the changes can occur fast enough to keep pace with the fast changing conditions [43].
The cellular events underlying coral bleaching are very complex and yet only partially understood. There are three steps in the cascade: (1) reactive oxygen species (ROS) production, (2) cellular signaling triggering bleaching and (3) Symbiodinium expulsion, either by exocytosis, host cell detachment or host cell apoptosis (Figure 4) [43]. Some cells survive thanks to the activation of anti-apoptotic proteins and may eventually enable future tissue regeneration [44]•.
Bleaching events are a prime example of altered inter-partner conversation, leading to either the symbionts leaving their host or the host expelling them. Recently, in situ gene profiling, using DNA stress-gene microarrays, has led to the identification of early sub-lethal effects of environmental stressors on still healthy corals [45]. Detection of early indicators of the endangered state of corals may enable us to take action before the irreversible onset of the bleaching cascade.
Figure 4. Reactions leading to bleaching.
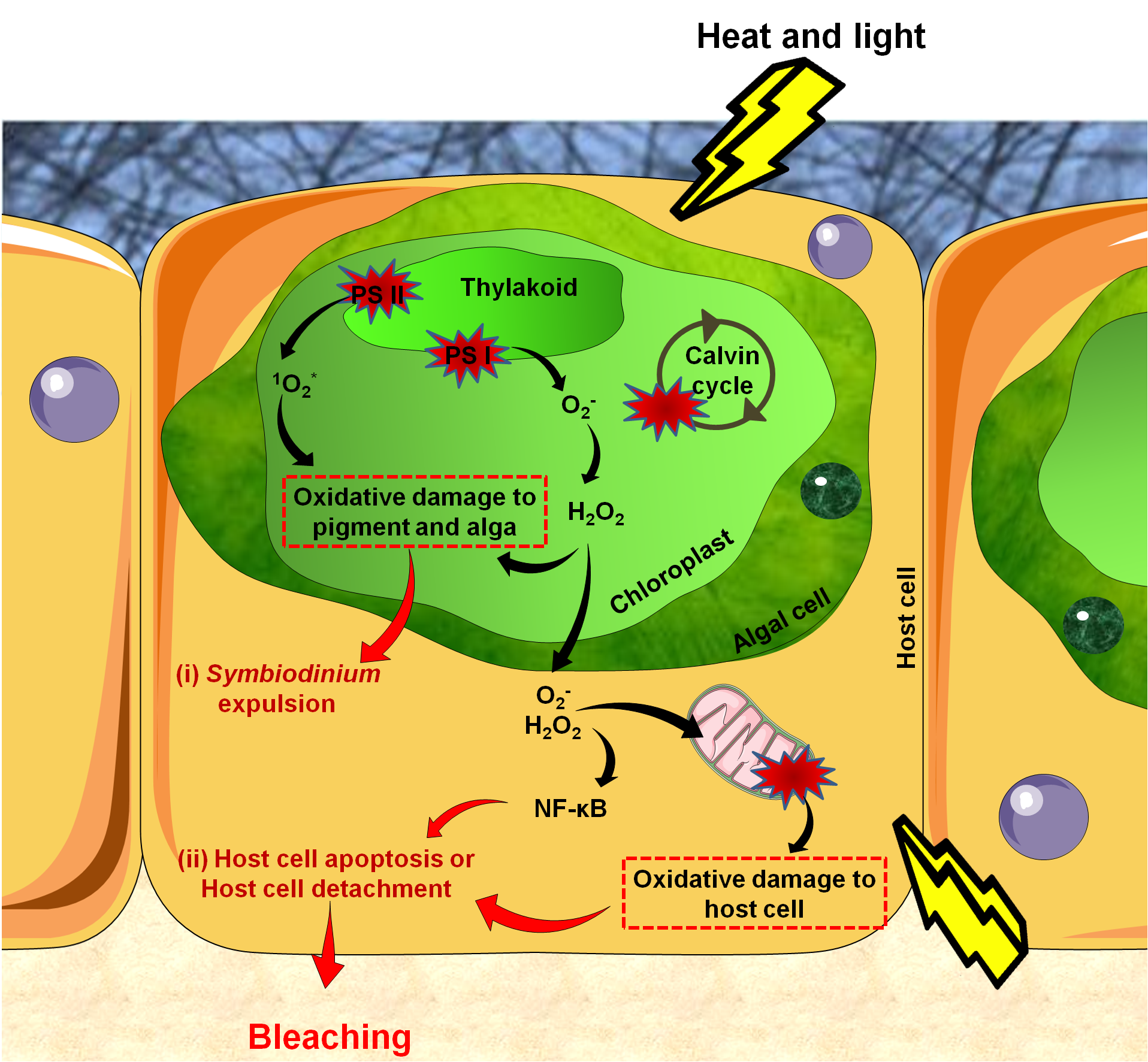
Reactive Oxygen Species (ROS) production starts in the alga due to damaged proteins in photosystem II (PS II), impairments of the Calvin cycle, and altered thylakoid membranes. Accumulated ROS then diffuse into host tissues causing damages to mitochondrial membranes, leading to ROS production in host tissues. This activates the innate immune response (NF-κB) which triggers apoptosis. Bleaching can be seen as the end of a controlled infection, with parasites signaling their presence by high ROS production.
Conclusion
In this review, we performed a comprehensive analysis of a particular type of symbiotic interaction: those which involve Symbiodinium . We firstly considered their phylogeny, in relation to that of their host, before studying the molecular basis of the interaction, ranging from metabolic exchanges to recognition mechanisms. Nevertheless, it is noteworthy that not only do Symbiodinium form symbiotic associations with marine invertebrate hosts, but also endosymbiotic cyanobacteria, or acoelomorph worms. And the “Holobiont”, referring to the host and all of the microorganisms taking part in the symbiosis, has a greater adaptive potential than the separate organisms, and could be considered as the unit of natural selection. Future research should be broaden to the entire complex network of organisms that interact together. As a whole, research in symbiosis will have rising importance in the decades to come. It will enrich our understanding of ecosystem functioning and biodiversity regulation, which has become critical at times of major environmental changes.
Acknowledgements
I wish to thank A. Moulding and S. R. Santos for allowing me to use their pictures, V. Goubard for her meticulous proofreading and V. Berteaux for introducing me to this subject and hosting me in her research team.
References and recommended reading
Papers of particular interest, published within the period of review, have been highlighted as:
● of special interest
●● of outstanding interest
1. Pettay DT, Wham DC, Pinzon JH, Lajeunesse TC: Genotypic diversity and spatial-temporal distribution of Symbiodinium clones in an abundant reef coral . Mol. Ecol. 2011, 20 :5197–5212. [ Full Paper ]
2. Stat M, Baker AC, Bourne DG, Correa AMS, Forsman Z, Huggett MJ, Pochon X, Skillings D, Toonen RJ, Van Oppen MJH, et al.: Chapter One - Molecular delineation of species in the coral holobiont. Advances in Marine Biology 2012. Edited by Michael Lesser. Academic Press; 2012:1–65.
3. Pochon X, Putnam HM, Burki F, Gates RD: Identifying and characterizing alternative molecular markers for the symbiotic and free-living dinoflagellate genus Symbiodinium . PLoS ONE 2012, 7 :e29816. [ Full Paper ]
4. Santos SR, Gutiérrez-Rodríguez C, Lasker HR, Coffroth MA: Symbiodinium sp. associations in the gorgonian Pseudopterogorgia elisabethae in the Bahamas: high levels of genetic variability and population structure in symbiotic dinoflagellates . Marine Biology 2003, 143 :111–120. [ Full Paper ]
5. Gómez-Cabrera M del C, Ortiz JC, Loh WKW, Ward S, Hoegh-Guldberg O: Acquisition of symbiotic dinoflagellates ( Symbiodinium ) by juveniles of the coral Acropora longicyathus . Coral Reefs 2008, 27 :219–226. [ Full Paper ]
6. Davy SK, Turner JR: Early development and acquisition of Zooxanthellae in the temperate symbiotic sea anemone Anthopleura ballii (Cocks) . Biol Bull 2003, 205 :66–72.
7. Fransolet D, Roberty S, Plumier J-C: Establishment of endosymbiosis: The case of cnidarians and Symbiodinium . Journal of Experimental Marine Biology and Ecology 2012, 420–421 :1–7. [ Full Paper ]
8. Detournay O, Schnitzler CE, Poole A, Weis
VM:
Regulation of cnidarian–dinoflagellate
mutualisms: Evidence that activation of a host TGFβ innate immune pathway
promotes tolerance of the symbiont
.
Developmental & Comparative Immunology
2012,
38
:525–537. [
Full Paper
]
● First paper to study the role of TGFβ immune pathway in cnidarians and its role in maintaining a
mutualistic association between hosts and symbionts.
9. Jimbo M, Suda Y, Koike K, Nakamura-Tsuruta
S, Kominami J, Kamei M, Hirabayashi J, Sakai R, Kamiya H:
Possible involvement of glycolipids in lectin-mediated
cellular transformation of symbiotic microalgae in corals
.
Journal of Experimental Marine Biology and
Ecology
2013,
439
:129–135. [
Full Paper
]
● In this paper,
the glycoconjugates
binding with SLL-2 and leading to algae morphological transformation are
identified on the Symbiodinium cell wall. Their role in host-symbiont crosstalk
is acknowledged and characterized.
10. Cumbo VR, Baird AH, Oppen MJH van: The promiscuous larvae: flexibility in the establishment of symbiosis in corals . Coral Reefs[date unknown], doi:10.1007/s00338-012-0951-7. [ Full Paper ]
11. Silverstein RN, Correa AMS, Baker AC: Specificity is rarely absolute in coral-algal symbiosis: implications for coral response to climate change . Proc. Biol. Sci. 2012, 279 :2609–2618. [ Full Paper ][ PubMed ]
12. Venn AA, Loram JE, Trapido-Rosenthal HG, Joyce DA, Douglas AE: Importance of time and place: patterns in abundance of Symbiodinium Clades A and B in the Tropical Sea Anemone Condylactis gigantea . Biol Bull 2008, 215 :243–252.
13. Finney JC, Pettay DT, Sampayo
EM, Warner ME, Oxenford HA, LaJeunesse TC:
The relative
significance of host–habitat, depth, and geography on the ecology, endemism, and
speciation of coral endosymbionts in the genus
Symbiodinium
.
Microb Ecol
2010,
60
:250–263. [
Full
Paper
]
●● The first paper performing an inclusive study of the factors affecting
Symbiodinium
distribution. Ecological, geographical and
bathymetrical factors, are combined with molecular analysis to identify the
pattern of host-symbiont repartition and address the question of specificity of
the interaction.
14. Yellowlees D, Rees TAV, Leggat W: Metabolic interactions between algal symbionts and invertebrate hosts . Plant, Cell & Environment 2008, 31 :679–694. [ Full Paper ]
15. Gordon BR, Leggat W:
Symbiodinium
—invertebrate symbioses and the role
of metabolomics
.
Marine Drugs
2010,
8
:2546–2568. [
Full
Paper
]
●● A complete overview of metabolic exchanges between hosts and symbionts,
with a characterization of the bilateral exchanges of nutritional metabolites,
of novel metabolic pathways that do not exist in either separate organism and
description of the most enigmatic identified metabolites.
16.
Godinot C, Houlbreque F, Grover R, Ferrier-Pagès C:
Coral uptake of inorganic
Phosphorus and Nitrogen negatively affected by simultaneous changes in
temperature and pH
.
PloS one
2011,
6
:e25024.
● The effect of rising temperature and decreasing pH
on nutrient uptake is precisely studied. The two factors are considered both
separately and combined to asses their relative impact.
17. Ganot P, Moya A, Magnone V, Allemand D, Furla P, Sabourault C: Adaptations to endosymbiosis in a cnidarian-dinoflagellate association: Differential gene expression and specific gene duplications . PLoS genetics 2011, 7 :e1002187.
18. Richier S, Furla P, Plantivaux A, Merle P-L, Allemand D: Symbiosis-induced adaptation to oxidative stress . J Exp Biol 2005, 208 :277–285. [ Full Paper ]
19. Rosic NN: Phylogenetic analysis of genes involved in mycosporine-like amino acid biosynthesis in symbiotic dinoflagellates . Appl Microbiol Biotechnol 2012, 94 :29–37. [ Full Paper ]
20. Sabourault C, Ganot P, Deleury E, Allemand D, Furla P: Comprehensive EST analysis of the symbiotic sea anemone, Anemonia viridis . BMC genomics 2009, 10 :333.
21. Rodriguez-Lanetty M, Phillips WS, Weis VM: Transcriptome analysis of a cnidarian-dinoflagellate mutualism reveals complex modulation of host gene expression . BMC Genomics 2006, 7 :23. [ Full Paper ][ PubMed ]
22. Hughes TP, Graham NAJ, Jackson JBC, Mumby PJ, Steneck RS: Rising to the challenge of sustaining coral reef resilience . Trends Ecol. Evol. 2010, 25 :633–642. [ Full Paper ]
23. Tambutté S, Holcomb M, Ferrier-Pagès C,
Reynaud S, Tambutté É, Zoccola D, Allemand D:
Coral biomineralization: From the gene to the environment
.
Journal of Experimental Marine Biology and
Ecology
2011,
408
:58–78. [
Full Paper
]
● A complete overview of the skeleton composition and structure, the
genes and mechanisms involved in biomineralization, and the
environmental factors impacting on the process.
24. Berkelmans R, Van Oppen MJH: The role of zooxanthellae in the thermal tolerance of corals: a “nugget of hope” for coral reefs in an era of climate change . Proc. Biol. Sci. 2006, 273 :2305–2312. [ Full Paper ][ PubMed ]
25. Shinzato C, Shoguchi E, Kawashima T, Hamada M, Hisata K, Tanaka M, Fujie M, Fujiwara M, Koyanagi R, Ikuta T, et al.: Using the Acropora digitifera genome to understand coral responses to environmental change . Nature 2011, 476 :320–323. [ Full Paper ]
26. Hofmann GE, O'Donnell MJ, Todgham AE: Using functional genomics to explore the effects of ocean acidification on calcifying marine organisms . Mar Ecol Prog Ser 2008, 373 :219–225.
27. Coffroth MA, Santos SR: Genetic diversity of symbiotic dinoflagellates in the genus Symbiodinium . Protist 2005, 156 :19–34. [ Full Paper ]
28. Aranda M, DeSalvo MK, Bayer T, Medina M, Voolstra CR: Evolutionary insights into scleractinian corals using comparative genomic hybridizations . BMC Genomics 2012, 13 :1–10. [ Full Paper ]
29. Pochon X, Montoya-Burgos JI, Stadelmann B, Pawlowski J: Molecular phylogeny, evolutionary rates, and divergence timing of the symbiotic dinoflagellate genus Symbiodinium . Molecular Phylogenetics and Evolution 2006, 38 :20–30. [ Full Paper ]
30. Pochon X, Gates RD:
A new
Symbiodinium
clade (Dinophyceae) from soritid
foraminifera in Hawai'i
.
Mol. Phylogenet. Evol.
2010,
56
:492–497. [
Full
Paper
][
PubMed
]
●● First paper to identify the previously unknown
Symbiodinium
clade I., from Foraminifera.
31. Kamezaki M, Higa M, Hirose M, Suda S, Reimer JD: Different zooxanthellae types in populations of the zoanthid Zoanthus sansibaricus along depth gradients in Okinawa, Japan . Marine Biodiversity 2012.
32. Wicks LC, Sampayo E, Gardner JPA, Davy SK: Local endemicity and high diversity characterise high-latitude coral– Symbiodinium partnerships . Coral Reefs 2010, 29 :989–1003. [ Full Paper ]
34. Loram JE, TRAPIDO-ROSENTHAL H, Douglas AE: Functional significance of genetically different symbiotic algae Symbiodinium in a coral reef symbiosis . Molecular Ecology 2007, 16 :4849–4857.
35. Biel KY, Gates RD, Muscatine L: Effects of free amino acids on the photosynthetic carbon metabolism of symbiotic dinoflagellates . Russ. J. Plant Physiol. 2007, 54 :171–183. [ Full Paper ]
36. Dimond J, Carrington E: Symbiosis regulation in a facultatively symbiotic temperate coral: zooxanthellae division and expulsion . Coral Reefs 2008, 27 :601–604.
37. Cumbo VR, Baird AH, Oppen MJH van: The promiscuous larvae: flexibility in the establishment of symbiosis in corals . Coral Reefs 2012, [ Full Paper ]
38. Castro-Sanguino C, Sánchez JA: Dispersal of Symbiodinium by the stoplight parrotfish Sparisoma viride . Biol. Lett. 2012, 8 :282–286. [ Full Paper ][ PubMed ]
39. Pasternak Z, Blasius B, Abelson A, Achituv Y: Host-finding behaviour and navigation capabilities of symbiotic zooxanthellae . Coral Reefs 2006, 25 :201–207.
40. Malcolm H, April H: The magnesium inhibition and arrested phagosome hypotheses: new perspectives on the evolution and ecology of Symbiodinium symbioses . Biol Rev Camb Philos Soc 2012, 87 :804–821. [ Full Paper ][ PubMed ]
41. Bertucci A, Tambutté É, Tambutté
S, Allemand D, Zoccola D:
Symbiosis-dependent gene expression in
coral–dinoflagellate association: cloning and characterization of a P-type
H+-ATPase gene
.
Proceedings of
the Royal Society B: Biological Sciences
2010,
277
:87–95.
● First paper to identify a symbiosis induced gene in zooxanthellae. This
gene codes for an H+- ATPase protein, crucial in
carbon concentrating mechanisms.
42. Yamashita H, Suzuki G, Hayashibara T, Koike K: Do corals select zooxanthellae by alternative discharge? Mar Biol 2011, 158 :87–100. [ Full Paper ]
43. Weis VM: Cellular mechanisms of Cnidarian bleaching: stress causes the collapse of symbiosis . J Exp Biol 2008, 211 :3059–3066. [ Full Paper ]
44. Ainsworth
T. D, Wasmund K, Ukani L, Seneca F, Yellowlees D, Miller D, Leggat W:
Defining the tipping point. A
complex cellular life/death balance in corals in response to
stress
.
Scientific Reports
2011,
1
. [
Full Paper
]
● A study of the apoptosis cascade
control in invertebrate-dinoflagellate symbiosis and its implication in
corals stress resistance.
45. Edge SE, Shearer TL, Morgan MB, Snell TW: Sub-lethal coral stress: Detecting molecular responses of coral populations to environmental conditions over space and time . Aquatic Toxicology 2012 , [ Full Paper ]