The Nile crocodile, a new model for investigating heterodonty and dental continuous renewal in vertebrates
01 - 10 - 2012
Abstract
Dental patterns in vertebrates range from absence of teeth to multiple sets of teeth that are replaced throughout life. Despite this great variation, most of our understanding of tooth development is derived from studies on just a few model organisms. The common model is mammalian, but during evolution the capacity for tooth replacement has been reduced in mammals. Therefore, replacement studies need to be extended to reptiles. Here I introduce the Nile Crocodile, Crocodylus niloticus , as an excellent model in which to study the molecular basis for early dental specification and, most importantly, for tooth replacement. I review studies on Crocodilians tooth organogenesis, which is regulated by an intricate network of epithelial and mesenchymal signaling during all steps of development. The dental hard tissues, dentin, enamel and cementum, are formed by unique cell types whose differentiation is intimately linked with morphogenesis, so I review the distinctive molecular patterning of the dental lamina. The simple architecture of the reptilian enamel organ is contrasted with the more complex, mammalian tooth bud and I discuss whether or not there is an enamel knot in reptilian teeth. Bioengineering of human teeth requires understanding of the mechanisms of normal tooth development, which I study here in a new animal model. Clinical tooth regeneration is depending on a better understanding of the epithelial-mesenchymal interactions during morphogenesis, and the process of multiple replacement as seen in reptiles.
Table of Contents
- Introduction
- The Crocodilian position regarding tooth evolution
- The appearance of tooth and jaw in Crocodilians
- Tooth initiation and formation in Crocodylus niloticus
- Tooth replacement in Crocodilians
- Molecular studies on dental development and replacement in mammals and squamate
- Conclusion and Perspectives
- References and recommended reading
Introduction
Acquisition of true teeth are one important innovation of the vertebrates and most of vertebrate species continuously replace lost teeth throughout their life. Teeth are also highly mineralized organs, which makes them the most important element in the fossil record of vertebrates. Therefore, teeth are an excellent evo-devo model, because we can as well study the dental development as the evolution of these structures. Fish, amphibians and reptiles display polyphyodonty, which means that they develop multiple sets of teeth, whereas most mammals form at most two generations (diphyodonty). The ability to ever replace the dentition was progressively lost during the transition from reptiles to mammals, in the frame of the evolution of Synapsida [1]. Although molecular mechanisms of tooth ever replacement in vertebrates are up to now poorly understood, this area constitutes a crucial challenge for evolutionary developmental biology because of the numerous applications it can bring in human tooth engineering.
In order to further investigate dental development and succession, I suggest using the Nile crocodile, Crocodylus niloticus as a model. It will allow us to study a continuous replacement, which is not possible in the common mammalian model (i.e. the mouse). The crocodile was chosen because it is interestingly placed in the vertebrate phylogeny between amphibians and mammals as seen in Figure 1. As reptilians are more closely linked to mammals than fish, it would be easier to understand why continuous renewal disappeared in mammals. It was also chosen because it is the closest living outgroup of birds [2], and could help understanding the loss of teeth in modern birds. Only few is known about the crocodile genome, we could thus take the opportunity to gather data needed to assess a new model animal. Our partnership with La Ferme aux Crocodiles made it possible to work on an important number of individuals.
Figure 1. Vertebrate phylogeny emphasizing the Crocodilians.
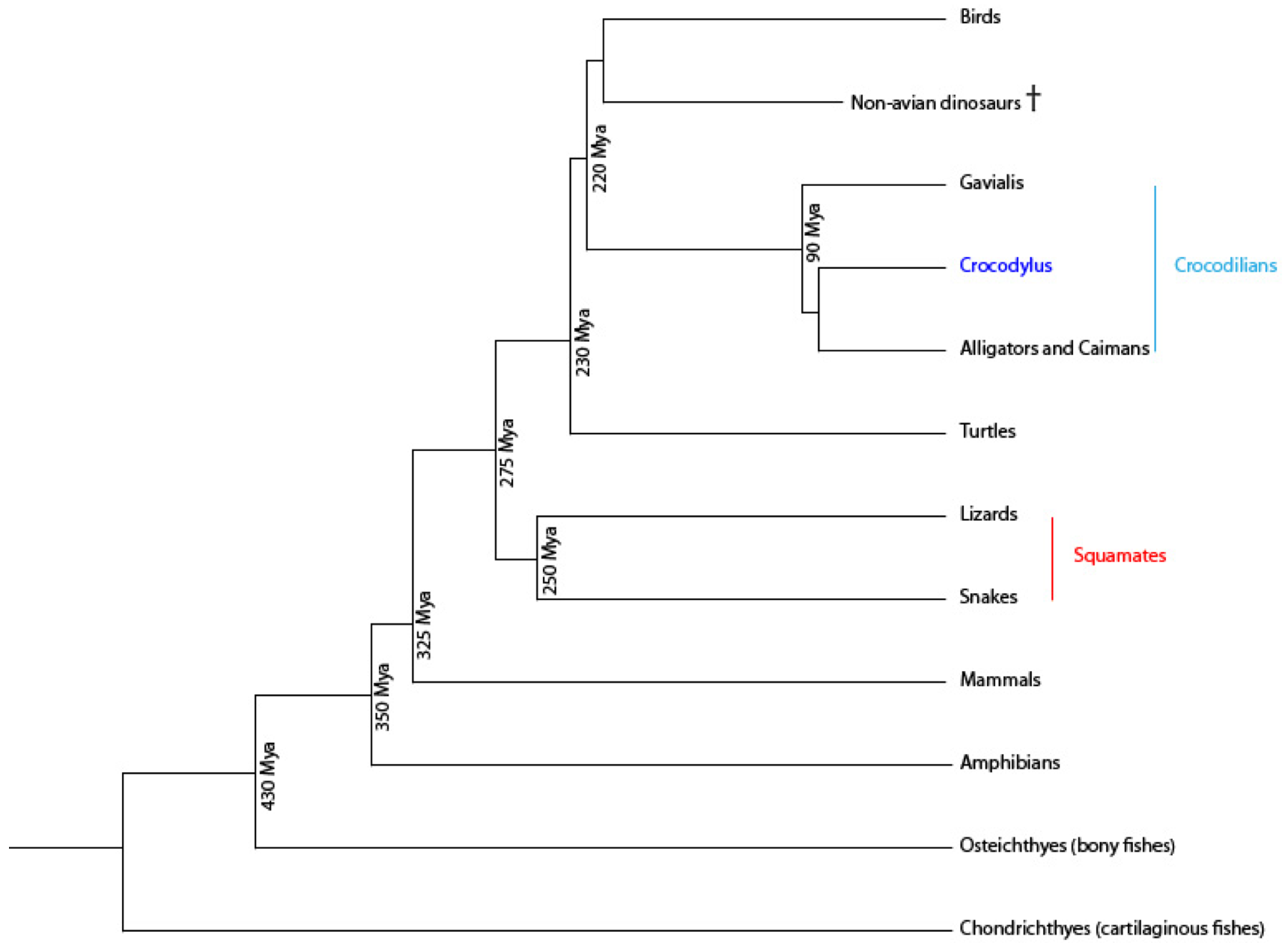
Approximative times of divergence are based respectively on the sequencing of Crocodilian genomes of three species: the American alligator ( Alligator mississippiensis ), the saltwater crocodile ( Crocodylus porosus ) and the Indian gharial ( Gavialis gangeticus ) [5]; as well as on extinct reptile studies [6]. Crocodilians are the best extant outgroup for comparative analysis of avian genomes.
Different models of tooth replacement in reptiles were proposed [3] and new data on Crocodylus niloticus will enable to evaluate how accurate they are. The study of dental replacement waves can be addressed using genetic tools. As they are well-known in the laboratory mouse, odontogenic structures in crocodile can be compared with equivalent ones in mouse. To characterize the dental development in crocodile embryo, a good understanding of gene expression in the tooth germ is needed, which is made possible by the recent discoveries about mammal and squamate tooth engineering.
The Crocodilian position regarding tooth evolution
As teeth constitute a direct survival factor through feeding, they were highly selected during vertebrate evolution. Even if variability is observed, jaw shape, tooth structure and composition stayed quite stable despite faunal and environmental changes [4]. It is thus interesting to compare teeth among vertebrates, and insofar Crocodilians constitute a good base for comparison.
Odontogenesis in vertebrates comprises oral epithelium–mesenchyme interactions during which the tooth germ passes through successive histo-morphological stages (i.e. lamina, bud, cap and bell stages) before tooth mineralization. In birds, the loss of teeth could be explained by the formation of a keratinized epithelium, the ramphoteca [2], which makes the epithelium–mesenchyme interactions impossible. Tooth rudiments were observed in embryos of talpid2 ( ta2 ) mutant chickens, in which several genes that are necessary for odontogenesis are expressed. These rudiments only occur at the rostral tip of dentaries and premaxillaries. They display a very simple conical shape and are composed of dental mesenchyme and epithelium, and show a putative dentin matrix, but the rudiments lack dentin and enamel secretion. Interestingly, they look similar in development, morphology and position to the first-generation teeth of Crocodilians and other reptiles.
Teeth evolved from dermal appendages, and through evolution, the number of tooth decreased while their morphological complexity increased [7]. The examination of development in the fossil record as well as evo-devo studies on living species are requisite for the study of life history evolution and odontogenetic mechanisms. Paleo-evo-devo permits developmental pathways to be extended into the past, constraining hypotheses of developmental evolution in ways that cannot be predicted by patterns observed from extant taxa alone [8]. Genetic investigations of odontogenesis are also needed to understand the phenotypic changes in animal lineages. The gain and loss of regulatory elements throughout vertebrate evolution and the changes in transcription factors, developmental genes and near extracellular signaling genes must be studied to characterize the evolutionary changes in tooth formation and replacement [9]. Besides, dental data play an important role in evolutionary and taxonomic theories.
In Crocodilians, the prenatal development represents a very interesting model for comparative embryological studies [10]. Such studies could help elucidating developmental mechanisms implicated during reptile–mammal evolutionary transition. As the speed of development of Crocodilians is very sensitive to developmental conditions, mainly temperature, the staging of embryos and fetuses is a very important prerequisite for correlating data between different developmental studies. In prenatal staging, the body weight can be considered as the most representative and complex parameter reflecting the overall growth of a whole embryo.
The appearance of tooth and jaw in Crocodilians
During the last century, a small number of anatomical studies were made on Crocodilians and can be taken as a basis for future work. Westergaard and Ferguson described precisely tooth initiation positions and sequences, pattern formation in Alligator mississippiensis embryos and juveniles [11-13]. These observations are very close to what can be observed in Crocodylus niloticus [14] and will therefore be considered as characteristic for Crocodilians.
In most species, as shown in Figure 2, anterior teeth are elongated and pointed, curved toward lingual side, with flattened lingual and buccal surfaces, displaying vertical ridges running from neck to tip and antero-posterior cutting edges. Their base is several times higher than the crown, which is about twice as high as the maximum diameter. The posterior teeth are short and squat, with rounded crowns and flattened bases which are not marquedly higher than crown. They present no cutting edges, aren't curved, and display irregular ridges. There is a gradual transition between these two types of shapes [15].
Figure 2. Appearance of two teeth of Crocodylus niloticus .
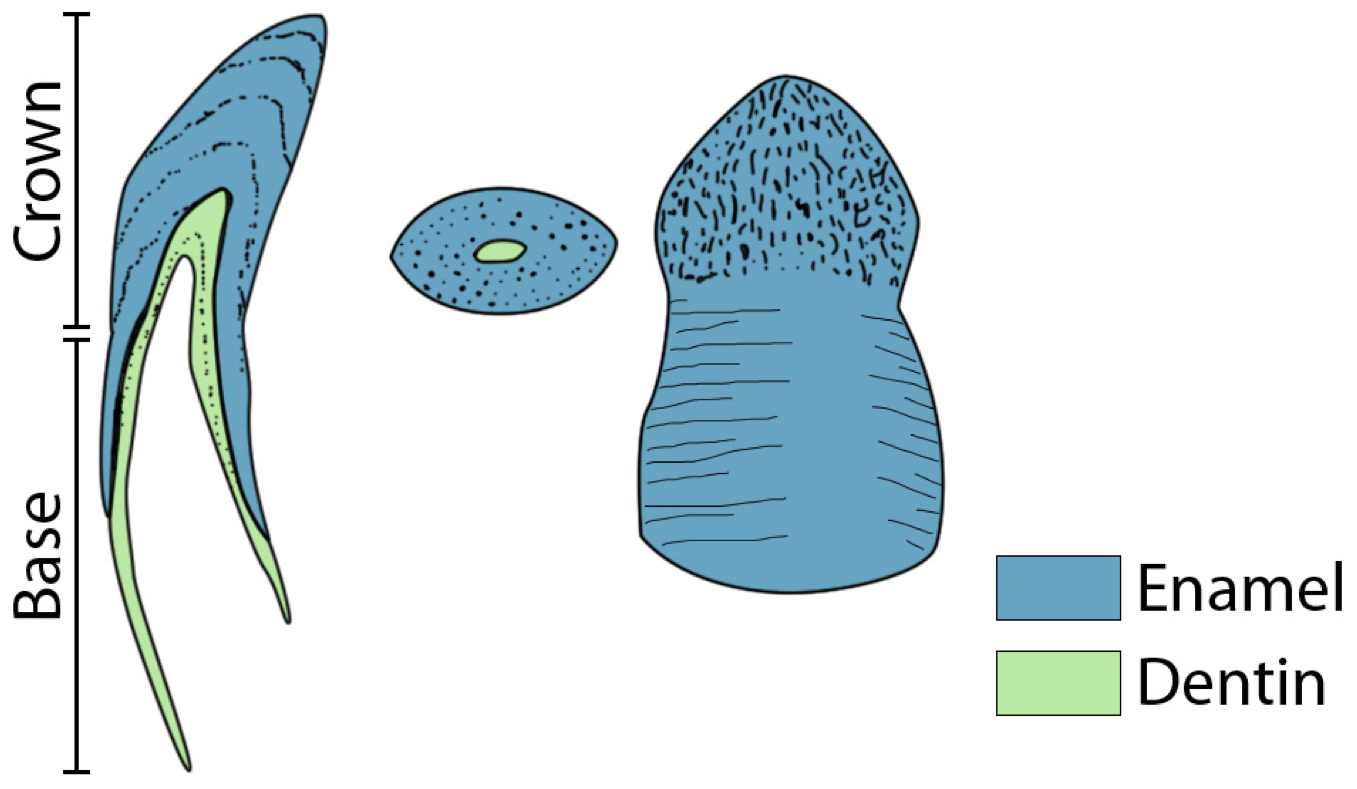
From left to right : sagittal cut of a front teeth, transversal cut of a back teeth and front view of a back teeth. The base constitutes the part of the teeth which isn't visible, but doesn't match the physiological root which is smaller. (Adapted from [15]).
Crown and base are separated by a more or less distinct neck or constriction. The base of the teeth is thin-walled and presents a wide open pulp chamber; the crown is heavy-walled and may contain little or no pulp. Usually one or two fangs appear in each jaw, fitting into a notch or pit in the opposite jaw. Crocodilians present a thecodont implantation, also called “socket-tooth”: teeth are set in alveoles in the jawbones, but in younger individuals, the posterior teeth are set in deep, relatively unconstricted grooves, which later become divided by inter-dental septa. This process is progressive from front to back. There is also presence of a peridontal membrane with a tooth-alveolus relation similar to mammals. A canal is going through the dental and the maxillary bones so that blood vessels and nerves pass near to the crypt where the replacement tooth forms. Young replacement teeth are still attached to the dental lamina, a plate of invaginated oral epithelium and divided in clumps of cells, one clump per alveolus. The early tooth development happens within the crypt and the relative age of a tooth can be estimated by its degree of eruption, but direct observation of a replacement tooth is impossible in Crocodilians without radiographs since the new tooth is hidden by the cap of its predecessor until a relatively late stage.
The general morphology of the Crocodylus niloticus oral cavity has been described and is the same in all adults: 15 teeth within the lower jaw, 18 within the upper [16]. In embryos and hatchlings, an inter-individual variability among animal at the same age of morphological and morphometrical characteristics can be observed, which decreases among animals of a similar body weight (irrespective of their age) [10]. Within the upper jaw, a separation between premaxillary and maxillary teeth can be observed, whereas in the lower jaw, teeth only grow on the dental bone. The biggest lower fang develops opposite to the premaxillary/maxillary junction, perhaps because less constraint is applied on them. Front and back teeth appear to be different, some authors distinguish some incisors (3 in lower jaw, 5 in upper), canines (5 in both) and molar (7 in lower, 8 in upper). Crocodylus niloticus are said to show “incipient heterodonty”: the first maxillary tooth is enlarged and divides dentition into incisors and cheek teeth. In some cases, “intrafamilial heterodonty” can be observed: the replacement tooth has a different shape than the functional one. Different theories try to explain these observations; tooth size and shape could be determined under influence of an ectomesenchymal substance in which cells migrate during odontogenesis, or different types of tooth clones (first only molar and incisors, then canines) could have appeared through evolution [14]. What is certain is that tooth initiation at a precise position appears to be controlled by the differential jaw growth.
Tooth initiation and formation in Crocodylus niloticus
The development of the dentition starts at specific positions in Crocodilian embryos being manifested by tooth placodes protruding into the oral cavity. Dental placodes have been related to the superficial, first-generation teeth that are abortive [13]. Tooth positions at later developmental stages of the Crocodylus niloticus are indicated by a regular series of protuberances on the free jaw margin.
The first formed tooth germ initiates in the anterior part of the jaw, but the most anterior teeth are not developing first. The remaining teeth were initiated in a rather random sequence, which rapidly changes to a regular alternation. The interstitial jaw growth allows new teeth to form, and in the back of the jaw teeth are first set in a furrow which only separates in individual holes after hatching. Dentition formation started by initiation of one tooth (dental determinant), localized condensation of cells in the epithelium (placode), then condensation of mesenchymal cells (dental papilla). The dental determinant initiates about the same time about first condensation of Meckel's cartilage. Then initiation spreads from the dental determinant backward and forward. In mammals, no regular alternation can be observed, and the formation of dentition starts from three dental determinants [17].
The dental lamina is a band of epithelial tissue seen in histological sections of a developing tooth [18]. It is formed when cells of the oral ectoderm proliferate faster than cells of other areas. This invagination of ectodermal tissues is the progenitor to the later ameloblasts and enamel while the ectomesenchyme is responsible for the dental papilla and later odontoblasts. Dental lamina is at first a continuous localized band of thickened epithelium which develops before the first tooth germs are visible, but as the epithelium over most of the dorsal surface of the lower jaw is already thickened. The lamina forms first in the front of the jaw and closes the dental furrow, and is later prolongated as a typical bilamenar dental lamina. The first formed embryonic teeth are poorly differentiated and lack enamel. In these teeth, the epithelium degenerates, nun-functional dentin rudiments persist and then become resorbed or shed. It would be interesting to identify some initiation, maintenance or death factors, especially in early teeth, because they would control the function of odontoblasts, whose biological function is the creation of dentin, and odontoclasts, which are osteoclasts associated with the absorption of the roots of deciduous teeth.
Tooth replacement in Crocodilians
Most vertebrates replace their teeth, and the replacement does not happen in a random sequence, as it would if it were only following tooth loss due to injuries. The waves of replacement are usually directed from back to front, and affect alternately numbered teeth, odd-numbered first, then even-numbered [3]. The distichy theory (Bolk, 1912), explains this fact by the presence of separate loci for development of odd- and even-numbered teeth, two odontostichos. One dentition is made of alternating elements of this two odontostichi and replaces itself in two distinct waves. Odd- and even-numbered waves are related to the sequence of tooth bud initiation.
But in Crocodilians, both back to front (caudad wave) and front to back (cephalad wave) replacement patterns can be observed, as well as instances with no apparent pattern whatsoever depending on the age of the studied animal. Replacement waves are caudad in younglings, then reverses in larger animals, around one year (skull length of 7 cm), to become cephalad [3]. In the oldest specimens, the irregularity of replacement patterns increasing strongly. This could be because the dental lamina becomes discontinuous in older crocodiles, but adjacent teeth keep a linked replacement pattern. Irregularity appears also because the teeth in the back are less replaced than those in the front, but this back/front difference is reduced in older animals. Figure 3 shows the dentition of different Crocodilians in which odd- or even-numbered waves can be recognized but it also appears that the pattern is highly irregular.
Figure 3. Comparison of Crocodilian dentition based on radiographs.
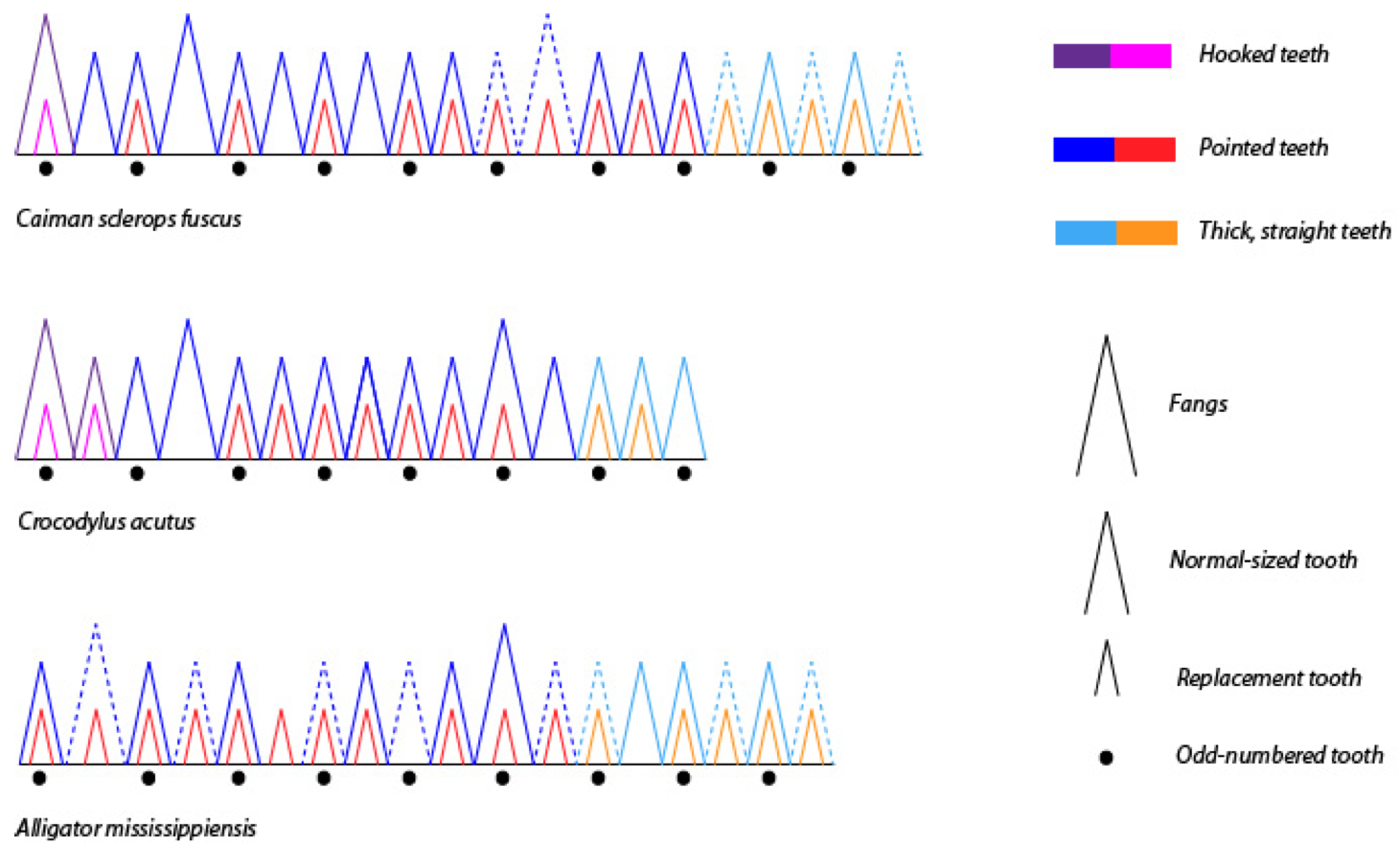
Lower jaw of young animals with different skull length: Caiman 10 cm ; Crocodile 8 cm and Caiman 4 cm. The difference of teeth form are shown in color, the pointed teeth are those in which the root is already highly resorbed and therefore near to falling off. (Adapted from [3]) .
The precise manner in which a tooth is replaced has also been described. A new tooth presents a short root when it erupts, which grows downwards into the socket. The pulp cavity in the crown begins to be occluded by further deposition of dentin. After the root has fully extended, it is resorbed at its base to make room for the next one. At last, the crown resorbs to and falls off while the replacement tooth grows larger [15]. So the form and condition of a functional tooth is related to size and condition of the replacement tooth. The tooth become higher with jaw growth, it could be because the root slowly erupts and becomes covered by a coat of enamel, but there is little support for this hypothesis. In general, every tooth has a replacement ready and a third tooth bud in formation. Pitman (1931) found 6 replacement teeth in the same socket for a Nile crocodile [15]. The new tooth erupts along the axis of the old one and takes its precise place in the jaw. Within the order Crocodilia, observations where made of tooth replacement ceasing in old individuals [3]. In mature animals, a great deposit of dentin within the pulp cavity is found.
The way the root grows downwards and then resorbs has been accurately described. The question is: Does the root of the functional tooth resorb to let the replacement tooth pass trough or because of the increasing pressure of the replacement tooth already growing inside the functional one? In fact, both mechanisms happen, one after the other. The first sign within the root is a small hole on the lingual side of the tooth, which then enlarges into a shape like an inverted V. The upward movement of the replacement tooth could be due to its increasing diameter, or to the next replacement tooth already growing beside and pushing it away. Anomalies are often seen, like two functional teeth placed in the same socket, which could be explained by a defect in the resorption of the root, and also parts of old root can be found, fully enclosed in new ones. Externally, the crown only changes because of wear and damage through use; but inside, occlusion of the pulp cavity by dentin deposits happens even after tooth eruption [15]. A particular white line, due to atypical calcification, could show a period of arrested development prior to eruption. There is a proportional increase between the length of tooth row and teeth diameter: At each generation, the successional tooth is at average bigger with 0.25 mm than the one before, so there must be a real important number of replacements during the crocodile's life. In certain lizards, replacement ceases when a certain size is reached [19].
Different theories explain the observed replacement patterns in Crocodilians. According to the Zahnreihe theory [3], basic units of dentition are controlled by chemical impulses which induce tooth initiation, and the direction of these waves depends on the spacing between Zahnreihen: if the distance is 2.0, alternate replacement occurs; if it is over 2.0 the waves are caudad and if it is under 2.0 the waves are cephalad. Along ageing of the animal, more Zahnreihen are added and the distance between them decreases which explains the switch in direction observed. But this theory, as well as Woederman's Odontostichi, do not match the data obtained in Alligator mississippiensis . In alligator, gecko and lizard, the pattern of regularly alternating tooth initiation is only present for a short time, no generalized theory upon regular alternation is credible.
Westergaard's tooth position theory is based on a chemical reaction-diffusion within the dental lamina, and is shown in Figure 4A. Tooth families are defined arbitrarily from separation by connective tissue [13]. The embryonic dental system appears to be evolutionarily very plastic: changes of jaw growth enable the creation or removal of tooth positions anywhere in the odontogenic area. Tooth families are constituted of one or more generations of teeth at the same place, the first generation tooth being replaced by the following generations. The decrease in the regularity of replacement may then be linked to the discontinuity of the dental lamina which is progressively interrupted by mesenchyme and losing contact with the oral epithelium. Initiation and eruption patterns of teeth seem to be correlated.
Osborn's theory of tooth clone says that dental initiation is due to growing clones of mesenchymal cells, after initial condensation occurs in the epithelium. The forward migration into the jaws of neural crest cells determines early initiation and tooth initiation starts in the anterior part of the jaw. According to this theory, the pattern of replacement mirrors the way the tooth germ grows [14]. It involves a zone of inhibition around each tooth, and initiation related to jaw growth. New primary teeth are added at a constant rate at the back of the jaw during growing. Secondary teeth appear in the interstitial space between primary one while this space grows, following an exponential increase in length with time. The inhibitory zone was modelised with a computer and matched the observation, in lower as well as in upper jaw. A tooth forms when the tissue escapes from an inhibitory zone rather than under the effect of molecules which initiate induction as seen in Figure 4B.
Figure 4. Comparison of Westergaard and Ferguson's tooth family model and Osborn's clone model.
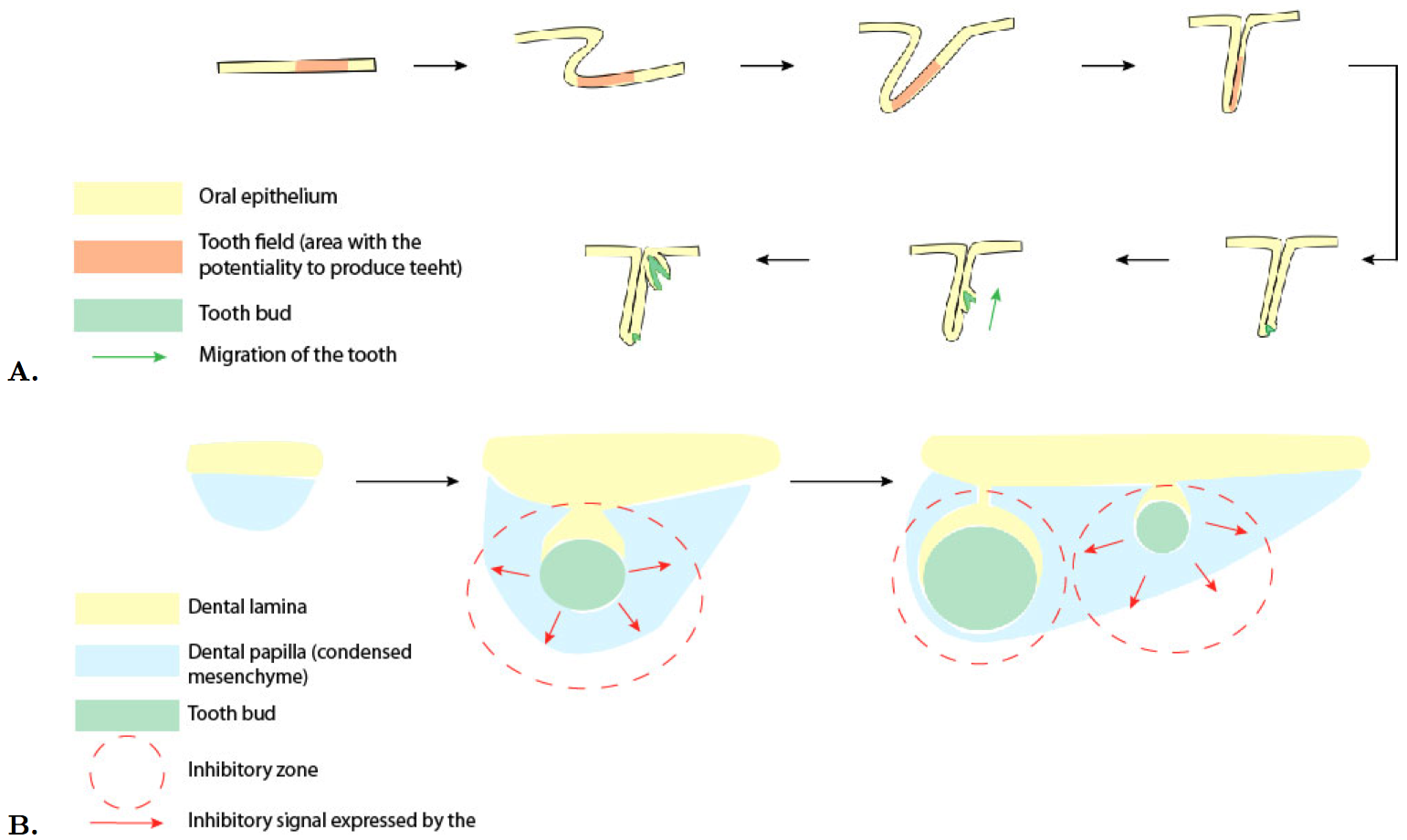
A. The tooth family model. The tooth field, the area with the potentiality to produce teeth invaginates. The first tooth anlage forms at it's free margin and migrates towards the surface during it's growth. As the first anlage reaches the surface, its replacement starts to develop [13]. (Adapted from Edmund [20]). B. The clone model. He proposed a quantification of the pattern of tooth initiation and a model based on the genes and molecules identified in mouse: FGF8 protein secreted by mandibular ectoderm induces underlying neural crest to transcribe the Pax9 gene. The surrounding epithelium secretes BMP4 (protein which prevents the Pax9 gene from being transcribed). Another protein, which needs to be identified, is transcribed by Pax9 gene and causes condensed cells to clump. The clump secretes a new protein which diffuses and inhibits any further cell clumping. The inhibitory zone contracts as the papilla becomes more surrounded by ectoderm. (Adapted from Osborn [14]).
Molecular studies on dental development and replacement in mammals and squamate
In embryology, the assumption is often made that similar events in different species are accomplished by similar processes [21]. Vertebrates are likely to share the tooth induction mechanism, pattern and sequence control. We now need to distinguish the biochemical or cellular mechanisms that happen in every teeth and those that control the pattern formation.
The study of tooth morphogenesis focuses often on the process of amelogenesis during tooth formation and the dichotomy between inner enamel epithelium growth and ameloblasts functions (secretion duration and rate). During tooth crown formation, relation between proliferation and differentiation is a key to understanding odontogenesis [22].
In the mouse model, synergetic and antagonistic effects of BMPs and FGFs are observed during odontogenesis. These factors first give positional information for initiation, then cells build up as an organ rudiment during morphogenesis and finally they differentiate and form an organ showing specific structures. The first sign of tooth development in mouse is the dental lamina, a thickening of the oral epithelium. A special structure, the enamel knot, secretes survival factors. It is a transient signaling center, which secretes SHH BMP2,- 4,-7 and FGF7,-9 and induces neighbor cells to produce enamel, but it also causes apoptosis in itself as shown in Figure 5A [23].
Genetic mutations leading to supernumerary teeth in mice and humans provide some insight into the molecular regulation of tooth replacement. A growing list of genes has been implicated in regulating tooth number. Continuous tooth generation in mouse, can be induced by Wnt/β-catenin signaling. The tooth bud forced to express stabilized β-catenin gives rise to dozens of teeth, which present a simplified unicuspid molar crown. This renewal process shows new signaling centers, the enamel knots, which bud off from the existing dental epithelium. This pattern can be reproduced with a computer model on tooth development. So the inhibition of this pathway in mammals could be the origin of their diphyodonty, and the capacity of renewal under Wnt influence is not lost in mammals [25]. Tooth organogenesis, lays under Wnt-Bmp control during sequential and reciprocal exchange of signaling molecules between the juxtaposed epithelial and mesenchymal tissues [26].
The genetic basis for forming replacement teeth in amniotes is poorly understood, in part because we do not have convenient animal models in which to study this process. Conventional mammalian models such as mice and rats only form a single generation of teeth. The multi-generational reptilian dental lamina appears to share some characteristics with some mammalian dentitions that have 2 generations (e.g., primates and Soricidae) in that replacement teeth appear to bud from the outer layers of previous generations [27]. Furthermore, reptiles are more closely related to mammals than are fish, the other main model in which tooth replacement is studied [28]. Therefore, developing reptilian models of tooth development will help to address the knowledge gaps in successional tooth formation.
In squamate, studies on continuous replacement where realized, and show that the patterning of snake dentition is dependent on Shh [27]. Cellular dynamics and molecular signalling in snake and lizard dentition show that tooth do not form from a separate germ but from a deep, ribbon-like lamina. Asymmetric cell divisions and extracellular matrix can be observed and suggest localized signaling. First, Shh is confined to the odontogenetic band and defines the position of the future dental lamina. Then Shh is expressed in inner enamel epithelium and stellate reticulum of tooth anlagen, but not in the outer enamel epithelium and it's derivative: another signal must be responsible for the replacement tooth formation. With Shh blocked, by using cycloplasmine, the dental lamina can't initiate and extend, and the directionality of this process is also affected. Also, blocking Shh leads to disruptions of the inner enamel epithelium. So Shh is controlling the depth of the dental lamina [29].
Figure 5. Gene expression and molecular signaling during tooth development in mouse (A) and snake (B).
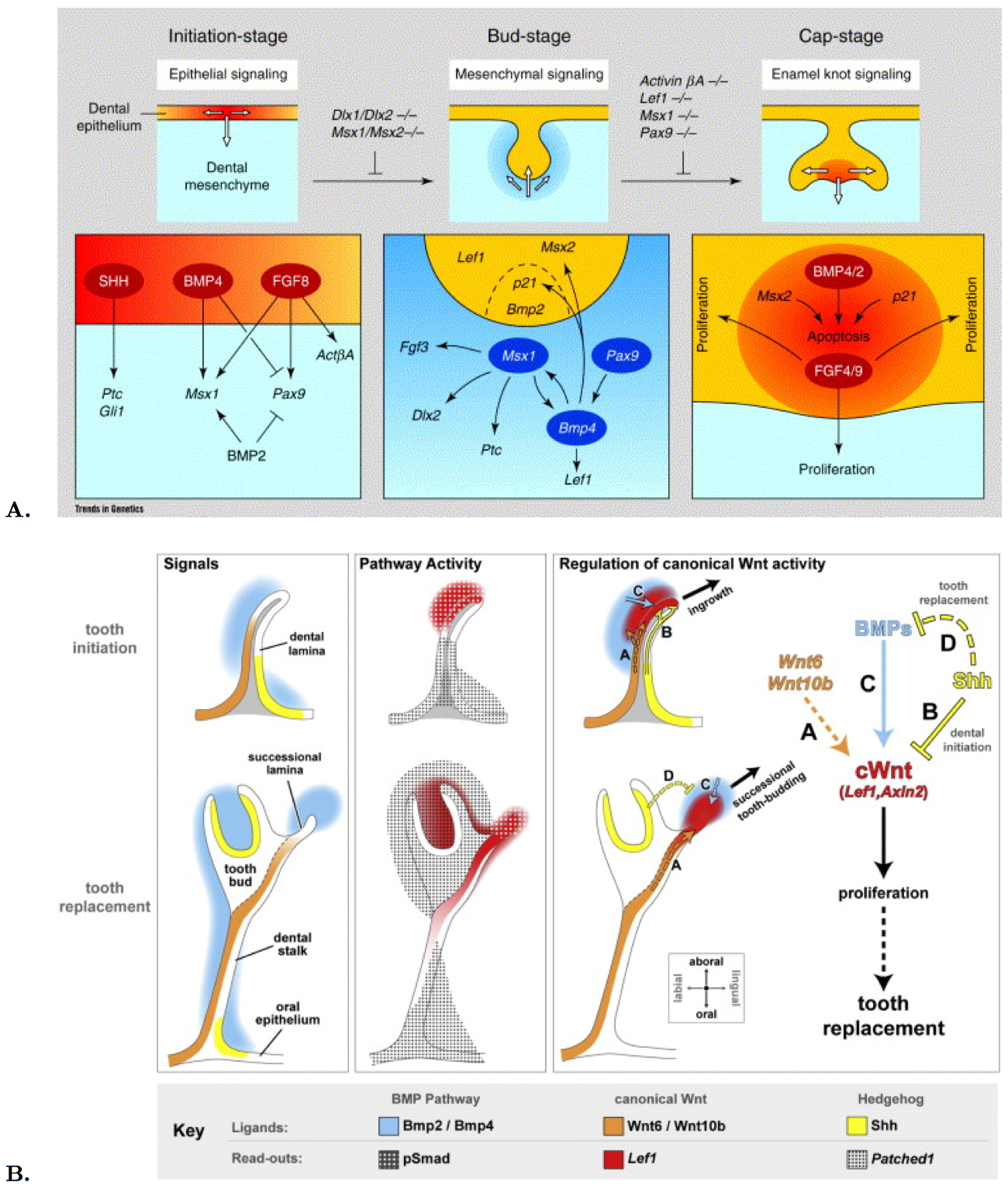
A. The upper panel shows three stages of early tooth morphogenesis, and white arrows indicate the direction of inductive events. The lower panel summarizes the current knowledge about the molecular pathways at each stage. (Reproduced from Peters [23]). B. The hedgehog, Bmp and canonical Wnt pathways are active during dental initiation (top row) and tooth replacement (bottom row). Gene expression is generally conserved between dental initiation and tooth replacement. Wnt ligand genes and Shh are expressed on opposite sides of the dental lamina, while Bmp genes are expressed throughout the dental mesenchyme, with particularly strong expression near the dental lamina tip and later the successional lamina. The Wnt and Shh pathways are active in complementary domains in the dental lamina throughout tooth development in snakes. (Reproduced from Handrigan and Richman [24]).
Dental stem cells and tooth replacement in lizard was also studied [30]. Wnt signaling appeared to regulate stem cell fate in the gecko dental epithelium [24]. Shh , Wnt and Bmp signaling network regulates tooth replacement in snakes as shown in Figure 5B. Wnt signaling regulates tooth number in mice and humans. Wnt genes are expressed in the dental lamina tip and surrounding mesenchyme, but Shh confines Wnt activity first in the dental epithelium under direct action, then during replacement stages by negatively regulating Bmp in the dental mesenchyme. Complementary domains of Wnt and Shh activity in the snake are found in the dental lamina and successional lamina. Expression of Wnt target genes correlates with areas of high cell proliferation, Wnt signaling induces cell proliferation in snake dental tissues. Mesenchymal Bmp can induce Wnt signalling in snake dental tissues. Epithelial Shh negatively regulates Bmp expression in the python dental mesenchyme. The spatial restriction of Wnt activity in the dental epithelium is a prerequisite for ordered tooth replacement in snakes. The lingual layer of the dental lamina houses a population of putative dental stem cells. These cells were identified on the basis of their slow cell-cycling times and their similarity to mammalian adult stem cells in terms of gene expression and regulation by the canonical Wnt pathway. They share two interesting characteristics in common with the gecko's putative dental stem cells: 1) they are non-proliferative; and 2) they are preferentially induced to proliferate in response to Wnt gain-of-function [30].
Epithelial stem cells have been identified in integumental structures such as hairs and continuously growing teeth of various rodents, and in the gut. The involvement of epithelial stem cells in the continuous tooth replacement that characterizes non-mammalian vertebrates, was studied in the example of zebrafish. Morphological observations of tooth renewal in the zebrafish were made and the similarities between molecular control of hair and tooth formation was noted, which opens perspectives for the work on Crocodilian teeth in relation to other epithelial products like skin appendages [28].
Conclusion and Perspectives
Crocodiles are usually thought to be homodont, which means that they develop a set of teeth displaying an overall same shape [14]. But preliminary examinations show the occurrence of fangs involving heterometric homodonty. The positioning of such teeth seems to be pre-determined: fangs occur at specific places in the jaw independently of the concerned dental generation. If fangs appear different from the other teeth, it is mostly a matter of size. Indeed, their shape is approximately the same as any other teeth. As their overall shape is almost identical than this of the other teeth, fangs should have the same morphogenesis. The hypothesis I make here is that these fangs could be simple teeth which are programmed to be bigger. I can hypothesize for further studies that fang size differenced could be related either to a different molecular expression, or to heterochronic expression of the same genes.
Furthermore some dental sectors display teeth with various incurvature degrees [31]. All these data raise the question of the molecular pathways involved in such light differences of size and shape. Besides this, it has been noted that crocodile embryos do not completely develop their first dental generation so that dental germs abort before turning into mineralized teeth [13]. Morphological investigations of this specific point associated with a study of molecular mechanisms could help us understanding specific mechanisms during tooth formation, for example the genes which are important for mineralization.
The crocodile has a continuous growth, so I wanted to verify to what extent was the Nile crocodile dentition following this positive allometry. Most results demonstrate that old embryos (over 50 days) and newborns already display the same number of teeth as the adults, the teeth being only smaller. Thus, the older Crocodylus niloticus grows, the bigger its jaws and teeth are, without a modification of the tooth number. In some jaws observed however, the last teeth were missing. This fact is likely to be caused by a smaller jaw bone. So an interesting issue is the genetic control of the total tooth number. The correlation between the apparition of new teeth and the length of the jaw will have to be studied in large developmental series.
In a future study, it would be important to find out which genes play an important role in the tooth replacement in Crocodylus niloticus . Genes like Shh [27], Wnt and Bmp [24] were already identified in other reptilians. But the most crucial challenge would be to find out which mechanisms allow the continuous dental replacement. I can hypothesize that epithelial stem cells should be involved in this replacement, like it is the case in fish. Stem cells were also identified in lizards [30]. Another interesting issue is the ability of the replacement tooth to go through the precedent tooth [15], therefore there must be a mechanism of degradation accompanying the apparition of the new tooth involving odontoclast control.
To find out in which way the replacement waves occur, we could continue this work on living animals by marking their teeth and following the modifications of the dentition through time. To better follow the replacement taking place, techniques such as fluorochrome sequential labeling can be used. Applied to mineralized tissues, this method recently proved to be feasible on teeth [32] and may provide a basis for further investigations of mineralization processes in teeth. It could allow the identification of structures like an enamel knot in the Crocodylus niloticus tooth, in addition to the study of genetics.
References and recommended reading
Papers of particular interest have been highlighted as:
● of special interest
●● of outstanding interest
1.Luo ZX, Kielan-Jaworowska Z and Richard LC:
Evolution of dental replacement in mammals.
Bulletin of Carnegie Museum of Natural History
2004, 36:159–175.
● The authors provided a review of dental replacement features in stem clades of mammals and a hypothetical outline for the evolution of replacement frequency, mode, and sequence in early mammalian evolution.
2.Louchart A and Viriot L: From snout to beak: the loss of teeth in birds. Trends in Ecology & Evolution 2011, 26:663–673.
3.Edmund AG:
Tooth replacement phenomenon in the lower vertebrates.
Contribution Life Science Division Royal Ontario Museum
1960, 52:1–190.
● The author studied the histology of dental replacement, especially in the order Crocodilia.
4.Anderson PSL, Friedman M, Martin DB and Rayfield EJ: Initial radiation of jaws demonstrated stability despite faunal and environmental change. Nature 2011, 476:206–209.
5.St John JA, Braun EL, Isberg SR, Miles LG, Chong AY, Gongora J, Dalzell P, Moran C, Bed'hom B, Abzhanov A et al.:
Sequencing three crocodilian genomes to illuminate the evolution of archosaurs and amniotes.
Genome Biology
2012, 13:415.
● The authors generated a phylogeny of reptiles based on genome studies.
6.Brochu CA, Parris DC, Grandstaff BS,
Denton RK and Gallagher WB:
A new species of
Borealosuchus
(Crocodyliformes, Eusuchia) from the Late
Cretaceous–early Paleogene of New Jersey.
Journal of Vertebrate Paleontology
2012, 1:105-116.
● The authors completed actual phylogenies with fossil data on past
Crocodilians.
7.Koussoulakou DS, Margaritis LH and Koussoulakos SL:
A curriculum vitae of teeth: evolution, generation, regeneration.
International Journal of Biological Sciences
2009, 5:226–243.
● The authors rewiewed current knowledge of the evolutionary relationships and the cellular and molecular mechanisms regulating the morphogenesis of a given tooth in its natural position.
8.Wilson LAB: The contribution of developmental palaeontology to extensions of evolutionary theory. Acta Zoologica 2011, 00:1–7.
9.Lowe CB, Kellis M, Siepel A, Raney BJ, Clamp M, Salama SR, Kingsley DM, Lindblad-Toh K and Haussler D: Three periods of regulatory innovation during vertebrate evolution. Science 2011, 333:1019–1024.
10.Peterka M, Sire JY, Hovorakova M,
Prochazka J, Fougeirol L, Peterkova R and Viriot L:
Prenatal
development of
Crocodylus niloticus niloticus
Laurenti,
1768.
Journal of Experimental Zoology. Part B, Molecular and Developmental Evolution
2010, 314:353–368.
● The authors aimed at establishing a background for future developmental
studies on
C. niloticus
: they documented and characterized
the prenatal development of the Nile crocodile on the basis of external
morphological criteria and head measures.
11.Westergaard B and Ferguson MW: Development of the dentition in Alligator mississippiensis : Early embryonic development in the lower jaw. Journal of Zoology 1986, 210:575–597.
12.Westergaard B and Ferguson MW: Development of the dentition in Alligator mississippiensis : Later development in the lower jaws of embryos, hatchlings and young juveniles. Journal of Zoology 1987, 212:191–222.
13.Westergaard B and Ferguson MW:
Development of the dentition in
Alligator
mississippiensis:
upper jaw dental and craniofacial development in
embryos, hatchlings, and young juveniles, with a comparison to lower jaw
development.
The American Journal of Anatomy
1990, 187:393–421.
●● The authors gathered and analyzed data on the tooth development and
replacement in
Alligator mississippiensis
.
14.Osborn JW:
Relationship between growth and the pattern of tooth initiation in alligator embryos.
Journal of Dental Research
1998, 77:1730–1738.
●● The author studied tooth initiation in the
Alligator
and showed that the sequence in which tooth positions are initiated in embryos may be more the result of the pattern in which cells escape from molecules that inhibit induction.
15.Poole DFG:
Notes on tooth replacement in the Nile crocodile,
Crocodylus
niloticus
.
Journal of Zoology
1960, 136:131–140.T
●● The author described the history and replacement of crocodile
teeth.
16.Putterill JF and Soley JT: General morphology of the oral cavity of the Nile crocodile, Crocodylus niloticus (Laurenti, 1768) I. palate and gingivae. The Onderstepoort Journal of Veterinary Research 2003, 70:281–297.
17.Osborn JW: The evolution of dentitions. The study of evolution suggests how the development of mammalian dentitions may be controlled. American Scientist 1973, 61:548–559.
18.Järvinen E, Tummers M and Thesleff I:
The role of the dental lamina in mammalian tooth replacement.
Journal of Experimental Zoology. Part B, Molecular and Developmental Evolution
2009, 312:281-291.
● The authors demonstrated that the replacement teeth forms from the dental lamina, which is intimately connected to the lingual aspect of the deciduous tooth enamel organ.
19.Handrigan GR and Richman JM:
Unicuspid and bicuspid tooth crown formation in squamates.
Journal of Experimental Zoology. Part B, Molecular and Developmental Evolution
2011, 316:598–608.
● The authors described the development of unicuspid and bicuspid teeth in squamate species
20.Edmund AG: Sequence and rate of tooth replacement in the Crocodilia. Royal Ontario Museum, University of Toronto 1962, 56:1-42.
21.Di-Poi N, Montoya-Burgos JI, Miller H, Pourquié O, Milinkovitch MC and Duboule D: Changes in Hox genes' structure and function during the evolution of the squamate body plan. Nature 2010, 464:99–103.
22.Keene H:
On heterochrony in heterodonty: A review of some problems in tooth morphogenesis and evolution.
American Journal of Physical Anthropology
1991, 34:251–282.
● The author demonstrated that, from a developmental point of view, it would seem appropriate to consider tooth size and enamel thickness as independent parameters of growth that contribute differentially to external crown dimensions.
23.Peters H and Balling R: Teeth.
Where and how to make them.
Trends in Genetics 1990
, 15:59–65.
●● The authors studied the developing tooth of the mouse as a model for organogenesis regulated by a series of interactions between morphologically distinct tissues. They generated information about the networks regulating reciprocal tissue interactions at the molecular level.
24.Handrigan GR and Richman JM:
A network of
Wnt
, hedgehog and
BMP
signaling pathways regulates tooth replacement in
snakes.
Developmental Biology
2010, 348:130–141.
●● The authors characterized
Wnt
signaling activity in
the dental tissues of the ball python,
Python regius
. They
suggested that hedgehog signaling confines
Wnt
activity in
the dental epithelium by direct planar repression and, during tooth replacement
stages, by negatively regulating BMP levels in the dental mesenchyme.
25.Järvinen E, Salazar-Ciudad I, Birchmeier W, Taketo MM, Jernvall J and Thesleff I: Continuous tooth generation in mouse is induced by activated epithelial Wnt/β-catenin signaling. Proceedings of the National Academy of Sciences of the United States of America 2006, 103:18627–18632.
26.O'Connell DJ, Ho JWK, Mammoto T, Turbe-Doan A, O'Connell JT, Haseley PS, Koo S, Kamiya N, Ingber DE, Park PJ et al.: A Wnt-Bmp feedback circuit controls intertissue signaling dynamics in tooth organogenesis. Science Signaling 2012, 5:ra4.
27.Buchtova M, Handrigan GR, Tucker AS, Lozanoff S, Town L, Fu K, Diewert VM, Wicking C and Richman JM: Initiation and patterning of the snake dentition are dependent on Sonic Hedgehog signaling. Developmental Biology 2008, 319:132–145.
28.Huysseune A and Thesleff I: Continuous tooth replacement: the possible involvement of epithelial stem cells. BioEssays: News and Reviews in Molecular, Cellular and Developmental Biology 2004, 26:665–671.
29.Handrigan GR and Richman JM: Autocrine and paracrine Shh signaling are necessary for tooth morphogenesis, but not tooth replacement in snakes and lizards (Squamata). Developmental Biology 2010, 337:171–186.
30.Handrigan GR, Leung KJ and Richman JM:
Identification of putative dental epithelial stem cells in a lizard with life-long tooth replacement.
Development
2010, 137:3545–3549.
● The authors defined a population of stem cells in the lingual dental lamina, the immediate descendents of which form the successional lamina and, ultimately, the replacement teeth.
31.Kieser JA, Klapsidis C, Law L
and Marion M:
Heterodonty and patterns of tooth replacement in
Crocodylus niloticus
.
Journal of Morphology
, 218:195–201.
●● The authors showed that there was a regular pattern of odontogenic
progression in
Corcodylus niloticus
, and that three
morphogenetic zones could be identified in each of the age classes: an incisor,
a canine, and a molar region. They thus concluded that the Nile crocodile is
heterorather than homodont.